Contents:
Quantum computer
Quantum computing is the holy grail of modern physics that has intrigued scientists ever since Richard Feynman proposed the first-ever model of a quantum computer in the 1980s. Even though both the world’s best universities and tech giants, like IBM, Google, and Intel, have significant investments in quantum computers, scientists have not developed a full-fledged quantum computer yet – but there already exist quantum programming languages based on simulation libraries.
Unlike conventional computers that are based on bits, which can be either 0 or 1, quantum computers rely on qubits, which can also be a superposition of both, meaning a 50/50 chance of the qubit being 0 or 1.
The probability of each state can be managed with the help of quantum logic gates, i.e., basic quantum circuits that convert the output data and increase the expected state to 99%.
One qubit can have two superposition states in a quantum system, while two qubits can have four, three qubits can have eight, and so on. Quantum computers need many hundreds of qubits to surpass the processing capacity of modern computers. In late 2022, IBM unveiled its Osprey 433-qubit quantum processor for IBM Quantum System Two.
Application: quantum computers will break the ground for a next-gen cryptography model. The existing algorithms are thought to be trustworthy until quantum computers grow strong enough to break the encryption system. The problem is that factorization – the decomposition of a prime number into its multipliers – is challenging for current computers. If the number and its multipliers are large, it will take a hundred years to decrypt them, whereas a quantum computer can do the task in a split second.
To that end, researchers are working on novel methods of data transmission and photonics-based coding techniques. They propose transferring photons over optical fiber as opposed to an electric signal via wires. In this case, data will be coded by the photon’s state, namely its vertical (0) or horizontal (1) polarization. Photons as well can achieve a superposition state, with two polarization states simultaneously. As this type of data can hardly be intercepted without exposing oneself, quantum encryption is considered to be more secure.
Quantum communications already exist: for instance, a quantum “highway” Moscow-St.Petersburg, with its length of approximately 700 kilometers, which has been developed by Russian Railways and ITMO’s Laboratory of Quantum Processes and Measurements.
Read also:
First-Ever “Quantum Call” Between Moscow and St. Petersburg Conducted
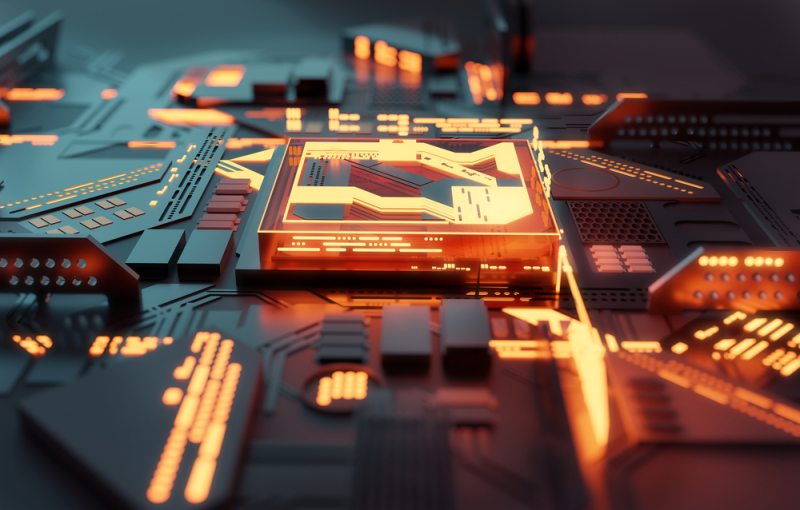
Credit: solarseven / photogenica.ru
Graphene
Graphene was discovered by Andre Geim and Konstantin Novoselov who received the Nobel Prize in Physics for this in 2010. Graphene is essentially a modification of carbon, like graphite or diamond, yet it consists of a single layer of atoms. These two-dimensional materials have excellent electrical and thermal conductivity, strength, and hydrophobicity. Inspired by graphene, the scientists also created silicene, phosphorene, and germanene.
Application: Although graphene cannot be utilized to build new objects, it is likely to usher in a technological revolution. Graphene's high conductivity paves the way for more powerful electronic devices, batteries, and solar cells, as well as ultrastrong materials and flexible displays.
Two-dimensional materials have long been on the radar of scientists, including those from ITMO’s School of Physics and Engineering – and a whole new team from a recently opened laboratory within ITMO’s Infochemistry Scientific Center.
Read also:
ITMO Scientists Suggest Cheap Way to Control Optical Signals With Halide Perovskites
ITMO’s New Lab To Develop Next-Gen Sensors and Associated Technologies
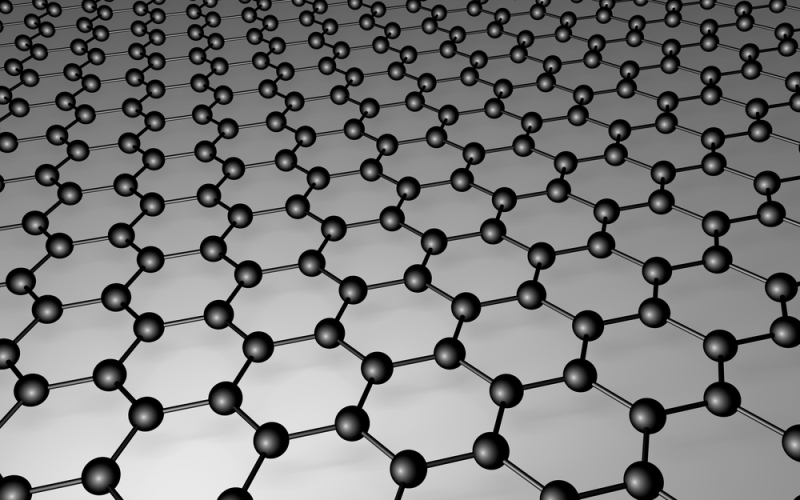
Graphene carbon network with atomic structure. Credit: Edgieus / photogenica.ru
Metamaterials
Metamaterials are another family of 2D materials that have a fundamentally different structure. They are an ensemble of identical nanostructures that display distinct optical properties defined by their shape. In metamaterials, the angle of refraction can reach 45-180 degrees, when normally it ranges between 0 and 90 degrees.
Therefore, specialists can quite accurately and freely regulate radiation within metamaterials, however, in a limited range: infrared, radio frequency – but yet not visible.
Application: first and foremost, metamaterials can be used to design optical computers, which will be incomparably faster since they would transport data using light rather than electric charges. They can also make ultrafast internet and communication systems possible.
Superlensing microscopy is yet another potential usage of metamaterials. The diffraction limit of current optical microscopes makes it impossible to identify objects that are smaller than a wavelength, which will no longer be a problem as it can be controlled in metamaterials.
And, finally, metamaterials can make an invisibility cloak a reality – once researchers embrace the visible range. ITMO researchers, for instance, have already created an invisible material based on dielectric nanoparticles in the anapole state that do not emit or transmit light and hence remain invisible to the human eye.
Read also:
Optical Computers Explained: Will They Replace the Regular Kind?
Invisible Material: Researchers Model Metasurfaces in Hybrid Anapole Modes for the First Time
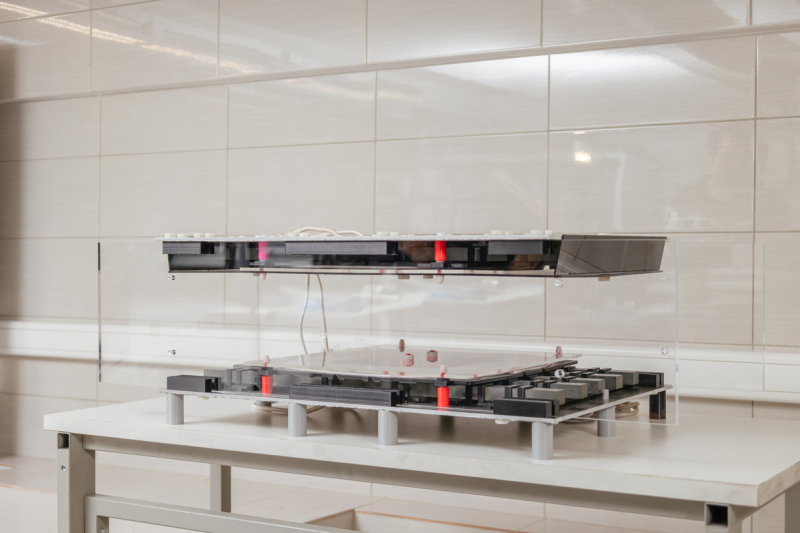
Researchers at ITMO’s School of Physics and Engineering have been pursuing wireless energy transmission for over five years. Their interest in the field arose from studying metamaterials and metasurfaces and applying their properties to enhance charging stations for medium-power mobile devices. The result was a prototype of a wireless energy transmission system for vehicles with a power output of 11 kW. Image provided by the School of Physics and Engineering, photo by Stepan Likhachev
Higgs boson
In terms of practical application, the Higgs boson is a useless particle – yet a key milestone in the pursuit of understanding the world.
Atoms consist of protons and neutrons, which, in turn, are made up of quarks, three in each. Quarks exchange energy – or more precisely nucleons – with one another, thus forming protons and neurons.
In actuality, science knows a lot more elementary particles, which are all laid out in the so-called Standard Model. This model assumes that particles have zero mass, which, however, does not correlate to reality. If zero-massed, particles display no inertia, which makes them chaotically travel around the universe at the speed of light, rather than interact and stick together with other particles as they do in reality.
Some researchers have suggested that there is a Higgs field permeating all of space where particles become ensnared and slow down, just like foam balls that can easily be blown away yet float on water.
Within this model, a Higgs field consists of those same bosons, which if ever discovered, will confirm the phenomenon. Researchers have been looking for the particle (which does not exist under normal conditions) for over 50 years now and even built the Large Hadron Collider in 2012.
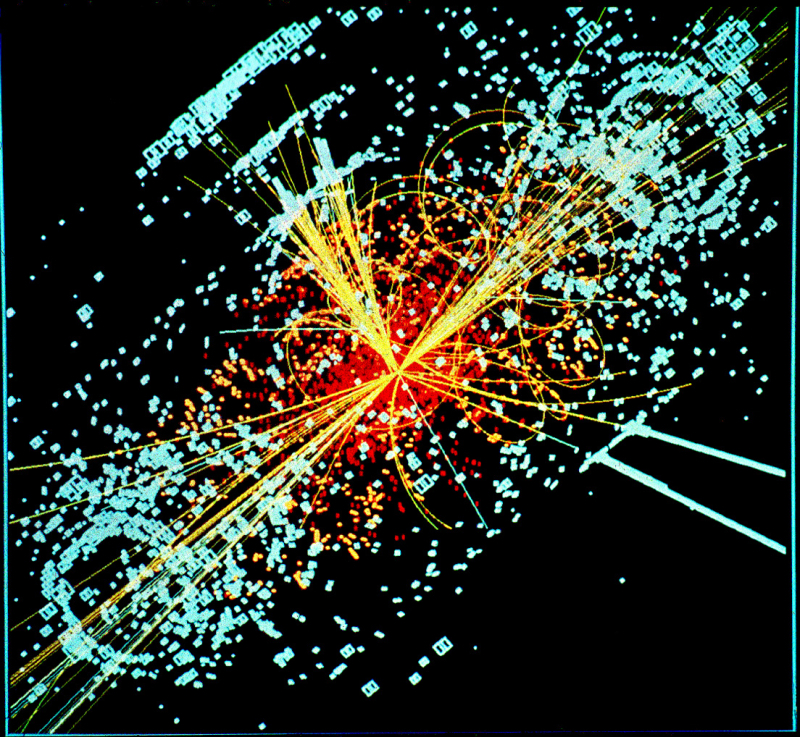
A simulation of a Higgs boson in a CMS particle detector. Credit: Lucas Taylor / CERN / Wikimedia Commons / CC BY-SA 3.0
Gravitational waves
Gravitational waves are a prediction of the theory of relativity by Albert Einstein who believe that gravity is the curvature of the universe caused by massive bodies. To understand how it works, think of a stretched fabric with a ball in the middle; other balls with less mass, thrown onto this fabric, will spiral down into the same hole, which was formed under the weight of the first ball.
Scientists have suggested that gravity travels in waves, like ripples in water. The problem is that planets and stars do not produce gravitational waves strong enough to be noticed. In contrast, black holes or two neutron stars, merging, create a massive disturbance. Although they fade out on their way to Earth, they can be detected using fairly sensitive gadgets.
The search for gravitational waves was conducted by a group of academics from some of the best universities in the world, including Lomonosov Moscow State University. They teamed up for the project LIGO back in 1980 to produce interferometers capable of detecting disturbances caused by merging black holes. As a result of their work on LIGO, Rainer Weiss, Barry Barish, and Kip Thorne all received the Nobel Prize.
Application: the finding of gravitational waves will have a huge influence on astronomy and astrophysics. While present electromagnetic radiation-based telescopes (optical, radiowave, infrared, ultraviolet, etc.) allow one to watch space objects, new ones will make it possible to "feel" them, as well.
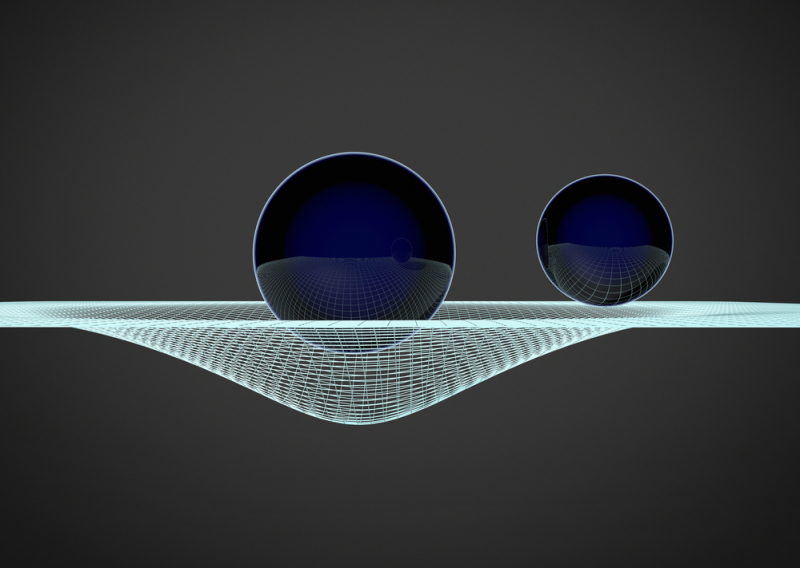
Gravitational waves. Credit: uberxoma / photogenica.ru
First image of a black hole
In 2019, scientists captured the first image of a black hole located 54 million light-years away from Earth using the Event Horizon Telescope (EHT), a large telescope array consisting of a global network of radio telescopes. The obtained image is, in fact, not a photograph of one, but a synthesized and processed image taken in the radio-frequency range.
It not only supports the theory of relativity, but it also proves the very existence of black holes, which turned out to be just as predicted by theoretical physicists – a “black hole” in the center, which does not emit any light due to gravity, and a glowing accretion disk around, created by particles that, similar to the rings of Jupiter, spin so fast that their friction produces colossal energy. The disk is not perfectly spherical but flattened due to the curvature of space and time.
Dmitry Pobedinsky’s lecture is part of the Physics Day’ program. A complete live stream of the talk can be found here (in Russian).