In the current age of digital technologies, regular users can hardly estimate the amount of information they produce and consume on a daily basis – hundreds of billions of emails, dozens of hours of videos per minute, and hundreds of thousands of search queries per second. The rapidly developing cloud storage technologies are making us consider leaving any physical storage devices in the past with magnetic hard disk drives (HDDs) gradually losing their share of the market. In recent years, they have been often substituted with solid state drives (SSDs). This, however, does not mean that HDDs are becoming obsolete.
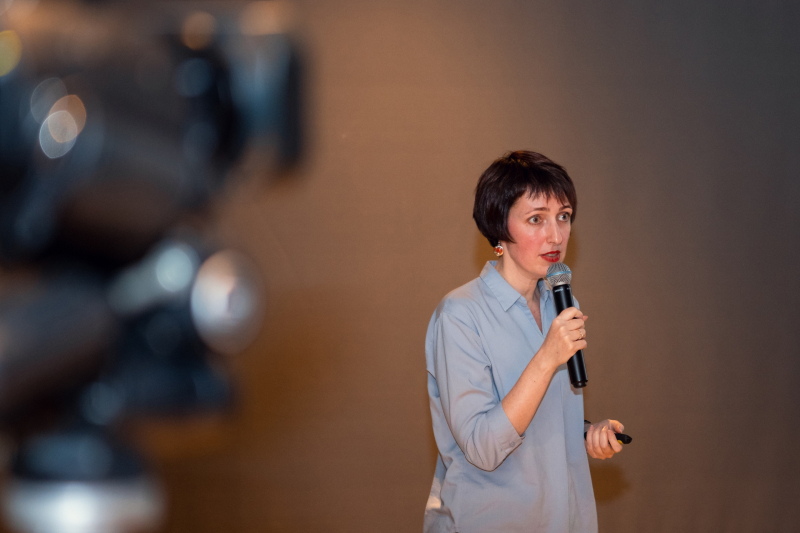
“Cloud services are actually based on the principles of magnetic storage, which remains to be the most cost- and energy efficient of its kind,” notes Alexandra Kalashnikova. “That’s why it requires further research. According to the latest forecasts, there will be HDDs with over 50 TB storage capacity on the market by 2026.”
What magnetic storage is made of and how it works
Let’s turn to physics for answers. It is “magnetic” because it requires a magnetic field to function. This field can be created by a coil, i.e. several whirls of a conductor with the electric current running through them. Another way to create the field is by using permanent magnets.
The nature of this phenomenon could only be explained with the development of quantum mechanics in the 20th century. The electron rotating around the core of an atom creates a tiny whirl of current, just like in a coil, and its spin becomes the source of a magnetic field. When atoms unite in groups, they can behave differently.
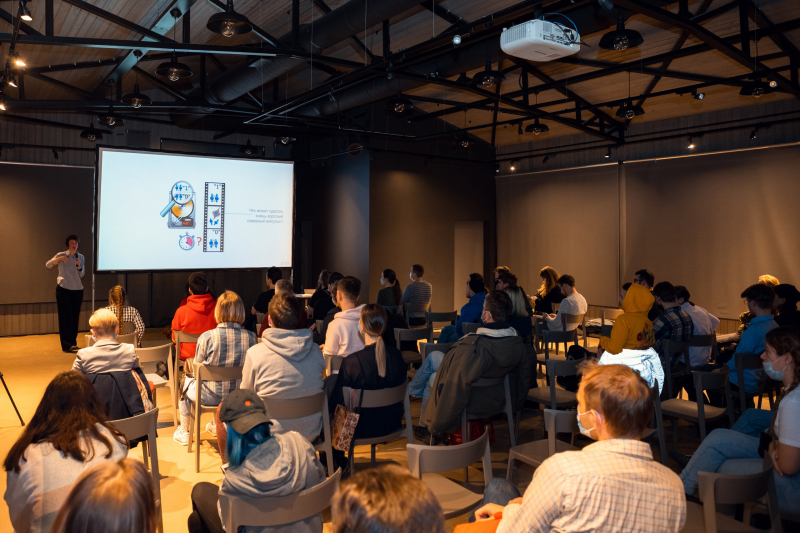
Magnetic storage research is particularly interested in a special type of material called ferromagnetics. In these materials, particles are located very close to each other and are organized in a certain way. They start “feeling” each other – and that serves as a basis for their interaction. As a result, the magnetic moments of these particles align parallel to each other. Their sum or magnetism can be measured in an actual experiment. With ferromagnetics it will equal a certain number, while in antiferromagnetics it will equal zero.
Magnetic bits
The magnetic anisotropy of ferromagnetics allows for storing the information in the conventional sequence of zeros and ones. This is because the magnetic moment can only face up (1) or down (0).
“Magnetic moments can be controlled with an external magnetic field: they will orient in accordance with the field and will maintain their direction after it is turned down,” comments Alexandra.
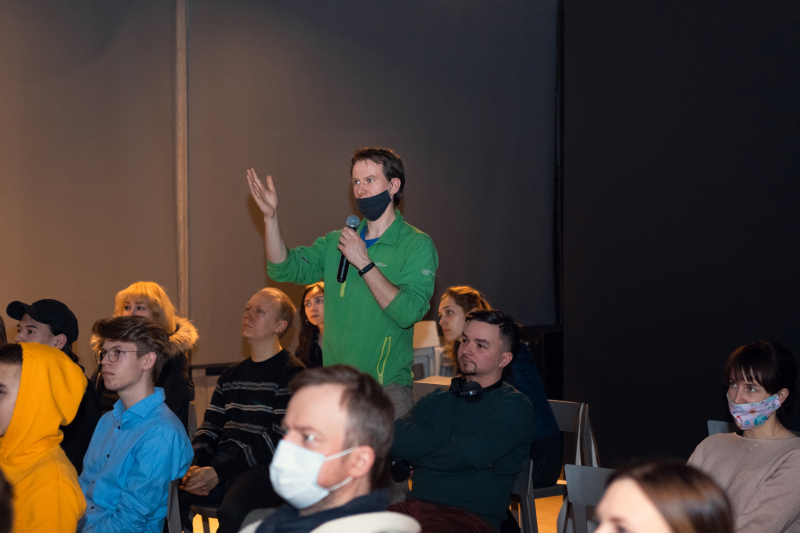
And this is how information is recorded on a magnetic drive. It then needs to be read from a region with an area measured in nanometers. A huge step in this field was brought by the discovery of giant magnetoresistance. It turned out that the orientation of magnetism of neighboring areas in relation to each other determines if there will be current in a circuit: if they are parallel to each other, the resistance is low and there is a current, and if they are facing different directions, it is vice versa.
Three issues of magnetic storage
When we store information, we need it to remain intact for extended periods of time. Mathematical estimations of the stability of magnetic storage suggest it will persist around 1,000 years. That’s a lot. But should we make bits just two times smaller, and the information will only be stored for two milliseconds. The attempt to accommodate this bit density by intensifying the magnetic field doesn’t work because of the decreased energy efficiency. But both researchers and regular users want to have it all: storage capacity, stability, and energy efficiency. How can we achieve it? By using light!
This is due to the fact that with higher temperatures ferromagnetics become softer which can be turned to good use. If we heat a small patch of the material with a laser impulse and at the same time apply an external magnetic field, we will perform heat-assisted magnetic recording (HAMR). This method requires a smaller magnetic field which means that both the energy efficiency and storage capacity of the drive will improve.
Faster, higher, stronger
Physicists, too, are not devoid of a sporting interest. The time it takes to turn the magnetic moment using HAMR is about 1 nanosecond, which is very slow. Back in 2004, researchers predicted that it won’t be possible to do it faster than 2 picoseconds which only presented a new a challenge to overcome!
“That’s just how it works in science: as soon as we have a strong claim that something is impossible, a lot of people strive to prove it wrong,” laughs Alexandra. “That is exactly what we are trying to do.”
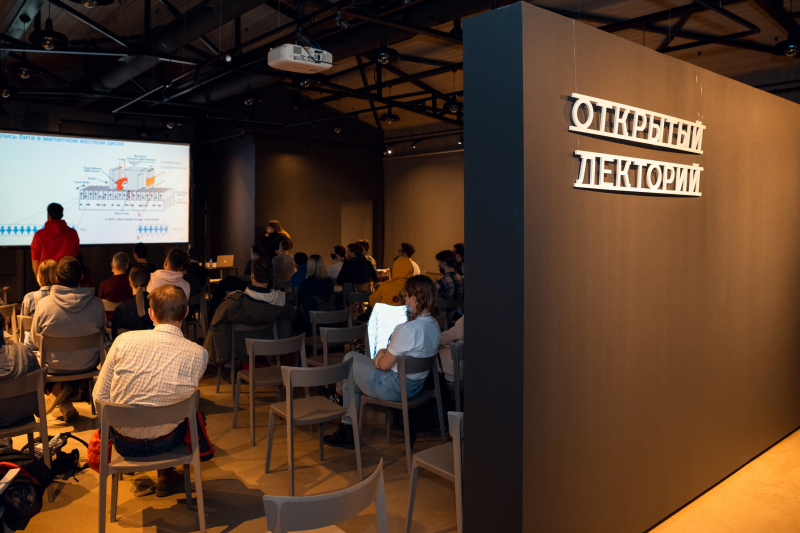
The problem can be solved with ultrashort femtosecond laser impulses. That’s a million times faster than in a normal HAMR which works at the speed of nanoseconds. A number of experiments demonstrated that magnetic media can change the properties of light and vice versa. As a result of such peculiar synergy of optics and magnetism we can observe an interesting effect: a heat-assisted magnetic recording without a magnetic field. This method takes into account every issue of information storage while also being superfast.
Exceed the limits
This project is currently more fundamental than applied and there are still several challenging but interesting issues to be solved before the researchers can move on to implementing the new method. For instance, it is necessary to get over the diffraction limit to provide a sufficiently high bit density. While this particular issue can already be solved thanks to advances in photonics, the challenge of turning ferromagnetics into antiferromagnetics remains to be overcome.
Written by Irina Vorontsova