Magnetic resonance microscopy
Magnetic resonance microscopy (MRM) is a type of magnetic resonance imaging that has especially high spatial resolution: the size of visualized structural elements of biological samples is often less than 100 microns. This method helps visualizing both internal organs and tissues as well as single cells. It is a useful instrument for scientists, as it offers the ability to generate digital sections of scanned samples that can be introduced in virtual 3D images. MRM is currently becoming a widespread visualization method in embryological research. For example, it helped creating the first 3D images of human embryos for the “Multi-Dimensional Human Embryo” project, a public database of three-dimensional embryo images.
The key goal of MRM is to create images of relatively small samples with the highest possible resolution. This is very important for analyzing living cells when studying their response to various excitations. For one, it is used for the function study of single neurons that are extracted from brains of laboratory animals. Studying such neurons makes it possible to shed light on how higher nervous activity works, which can in turn help to develop means for treating neurological diseases.
Several research teams from all over the world focus on projects in this field. For example, one such team works at the NeuroSpin research center in Paris. Its scientists study the human brain using various methods, MRI included. The center has both tomographs aimed at human brain study and the so-called pre-clinical tomographs with a higher level of field (up to 17T). Such tomographs have a tunnel diameter of only 9cm, and are used for studying laboratory animals and extracted parts of their brains.
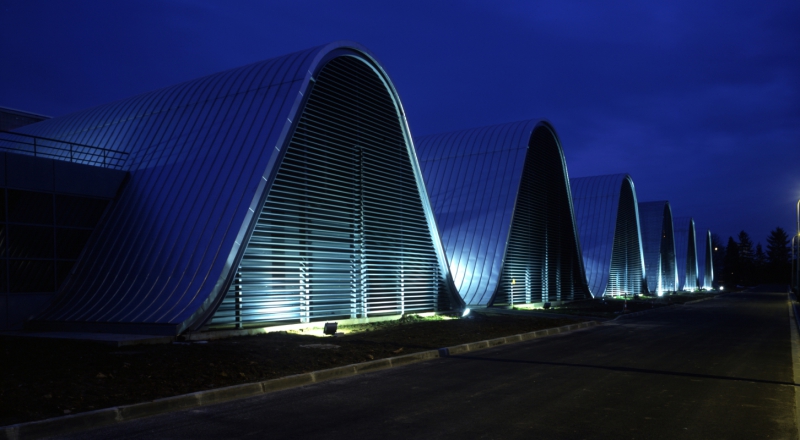
MRM is best conducted on high-field tomographs, as the higher the field is, the better is the signal-to-noise ratio, hence it takes less time to get an image with a better resolution.
Associated problems
In order to increase the image quality, researchers are interested in attaining the smallest size of a voxel (the basic element from which the image is created). But as we increase the resolution and decrease the voxel size, the signal-to-noise ratio also decreases. It would have seemed that we can infinitely increase the resolution, but that would increase the amount of noise, which can make the image undescriptive.
According to Stanislav Glybovski, one of the article’s authors and staff member of ITMO’s Faculty of Physics and Engineering, there are several approaches that can help to increase the signal-to-noise ratio. First of all, that would be elevating the magnet’s field. Pre-clinical tomographs with a level of field of 17T are systems that offer a resolution that is closest to today’s maximum. Still, this is also associated with several problems.
At 17T, a magnet becomes unstable. It is hard to attain stable operation, so such systems like the one at NeuroSpin are unique. Due to the unstable work of the magnet, scientists can’t scan big samples. For example, it is impossible to get a high-quality image of the whole body of a laboratory animal. You can only scan a small area, because within the borders of this area the magnetic field has homogeneous distribution (and thus the frequency to which the spins of hydrogen nuclei respond is also homogenous). If the field is not homogenous, then the frequency isn’t either, and the signals from the spins for the part of the sample will not fall into the receiver bandwidth.
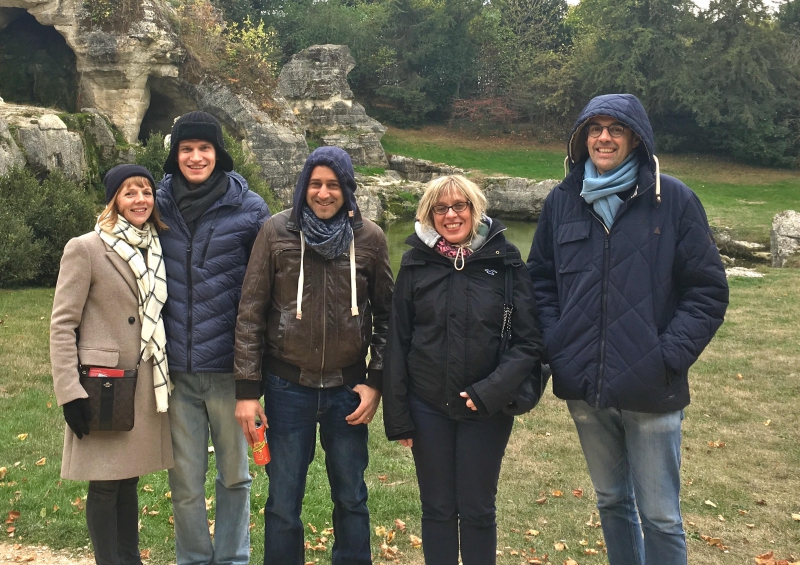
What’s more, the signal-to-noise ratio is also limited by the radio-frequency probes that are being used, and the properties of the receiver. The classical solutions used in 17T tomographs is copper solenoids as probes inside which the samples go. Still, copper’s conductivity is limited, which is why a portion of the useful signal dissipates within the probe and fails to reach the receiver. The other limitation is associated with the fact that the solenoid creates a quasi-static electrical field in addition to the magnetic one. This also leads to energy losses in the sample.
Proposed solution
A group of scientists from ITMO University, Institut Fresnel, Leiden University Medical Center and the NeuroSpin research center proposed replacing the solenoid with a dielectric probe created with the help of a unique composite material developed at Ceramics Jsc. The outer diameter of the new dielectric resonator is 18mm, the inner is 6mm. It is manufactured as a cylinder with a hole where the sample goes.
The research was a part of the collaboration on the M-Cube project (an international project conducted within Horizon-2020 program, you can read more about it here). Scientists from ITMO University made numerical calculation and conducted experimental research of the resonator. At Institut Fresnel, physicists Marine Moussu and Redha Abdeddaim developed a functional analytical model of both probes, the solenoid and the coil based on a dielectric resonator, which allowed to predict the possible limit of the signal-to-noise ratio for each of them, as well as define which dielectric is best for creating a probe that will exceed the possibilities of solenoids in terms of the signal-to-noise ratio.
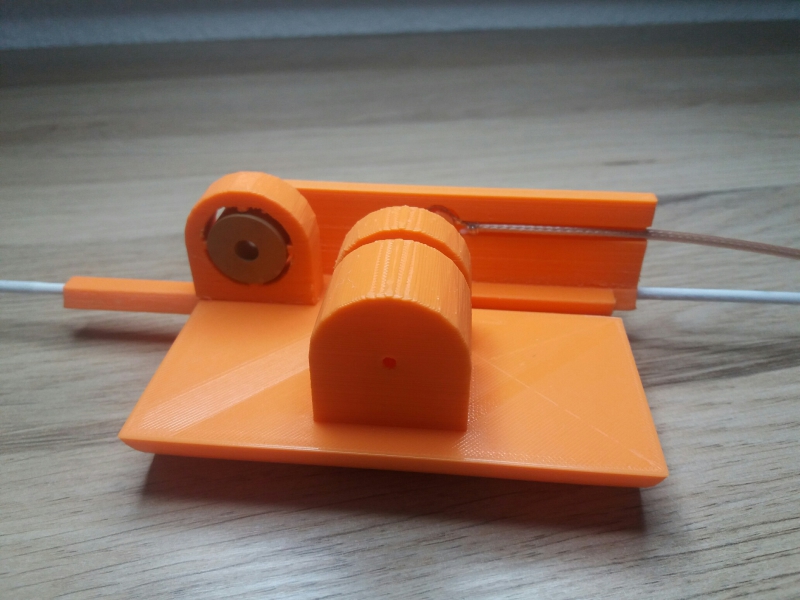
The scientists learned that two conditions have to be met in order to create such a dielectric probe. First of all, one needs a material with very high permittivity (an indicator that characterizes the molecular response of a material to external electric field). Every material has such a paroperty. For example, the permittivity of air is 1, while ceramics used for domestic needs has a permittivity of of the order of 10. For the purposes of creating a signal-to-noise ratio which is twice higher than that which was optimized for the solenoid probe, one has to use a material with a permittivity of about 500. Secondly, it has to have very low dissipation losses: a dielectric loss tangent of no more than 0.0008 at 730 MHz .
Material used for the resonator
Such high indications of permittivity and low dielectric losses are demonstrated only by specially synthesized ferrielectric materials in paraelectric field. Such materials are developed by specialists of Ceramics Jsc. Calculations showed that one of the company’s unique inventions is best suited for new resonators. Ceramics Jsc. has been collaborating with ITMO University for more than five years; what’s more, among its partners are some of the best laboratories and research centers of Europe and the USA.
“Modern ceramic materials are multicomponent, complex polycrystalline compounds. One can call them “smart materials” – materials with a targeted set of properties. We create new ceramic materials based on our experience as well as analysis of preliminary research conducted with the use of modern methods of physical and chemical analysis and metrology. We know how to control materials’ properties, and create ceramics for various purposes, most importantly for the field of electronic engineering. What’s more, we also create items from these materials,” comments Elizaveta Nenasheva, CEO of Ceramics Jsc.
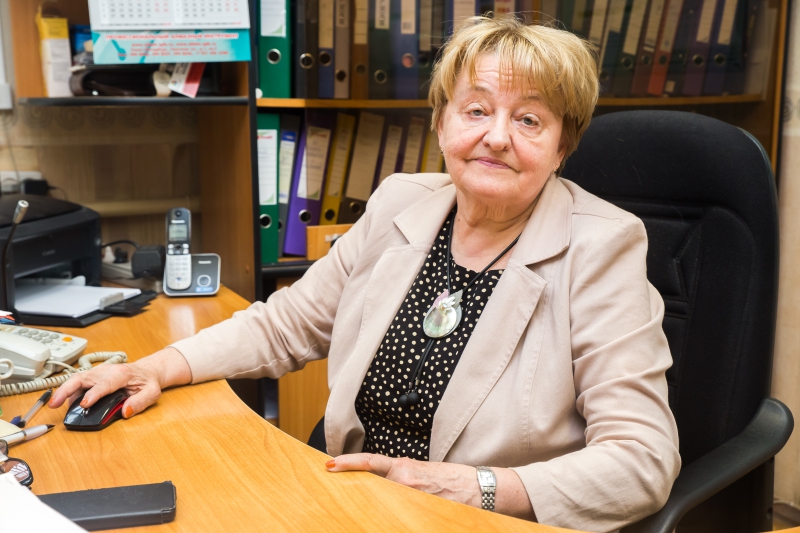
The key feature of the composite ceramic material that was used for the new probe, namely low dielectric losses at a relatively high permittivity, are associated with its unique composition and the technologies of its synthesis. Its base was developed in 2007 and patented in both Russia and the USA. According to Elizaveta Nenasheva, it has already found an application in microwave controlled devices for high-power accelerating systems developed in the US and got successfully tested for use in the main accelerator at CERN. And recently, the researchers found out that such materials are promising for MRI systems, as well.
“The opportunity to control permittivity is very important for a material used in devices controlled by electric fields, such as microwave filters, phase shifters, etc. Providing for a better control is often associated with a slight increase in dielectric losses in ferroelectric composite ceramics. Our material exceeds all existing counterparts in the totality of parameters. Then again, the absence of essential requirements to control characteristics in MRI systems allowed us to update the material’s formula and considerably decrease the dielectric losses in the bandwidth that MRI works in,” she adds.
The technology of ceramics manufacturing is a complex science-intensive process that involves several stages. It all begins with the process of mixing the basic components in the necessary proportion, then the material is synthesized, then it is regrounded, and the organic bond for hydroforming and isostatic molding of samples is introduced. Then, the samples are sintered in special electric ovens, and a radiographic inspection of their phase composition is carried out. Then, the samples are grinded into the necessary size, and their electrical characteristics in the required bandwidth are measured. For this purpose, the samples are metallized in advance. The final stage is controlling the exterior of the samples and packing them.
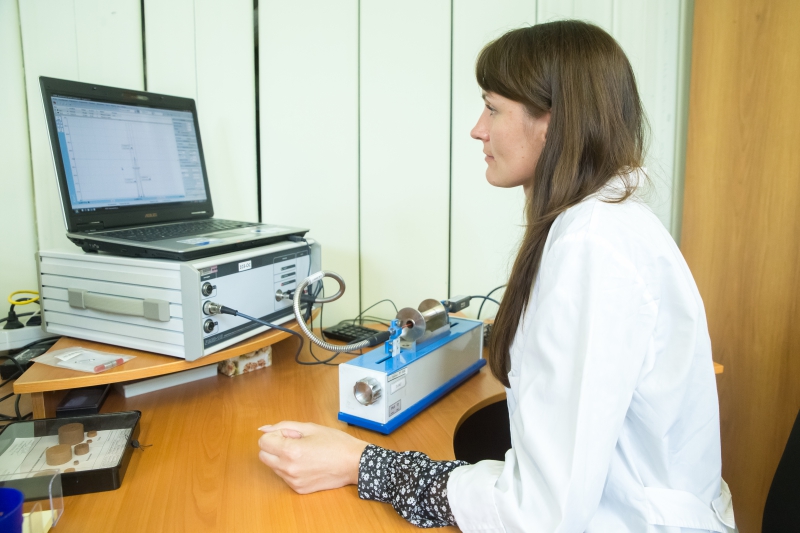
Experiments’ results
The new material was used to create a probe that was tested with a new high field tomograph Bruker with a field of 17T at the NeuroSpin research center. The probe was compared with a solenoid optimized for a cylindrical sample of 4mm in diameter and 12mm in length. The experiment showed a two-times increase in the signal-to-noise ratio when using a dielectric resonator.
“We’ve proved theoretically that this probe’s sensitivity is two times higher than that of a copper solenoid, the gold standard in MRI. Our experiments on various samples –stalks, small fruits and biological tissues – proved these theoretical results. The key advantage of the new device is an increase in sensitivity, which is essential for magnetic resonance microscopy,” comments Luisa Ciobanu, staff member of NeuroSpin and one of the article’s authors.
Stanislav Glybovski adds that the dielectric-based probe is also quite easy to use in comparison with the solenoid one. In order to attain the best signal-to-noise ratio, one usually has to choose the capacitance of the tuning circuit before every experiment, and constantly adjust the properties of the coil, as the frequency depends on the properties of the object under study. Using a dielectric resonator makes the process a lot simpler.
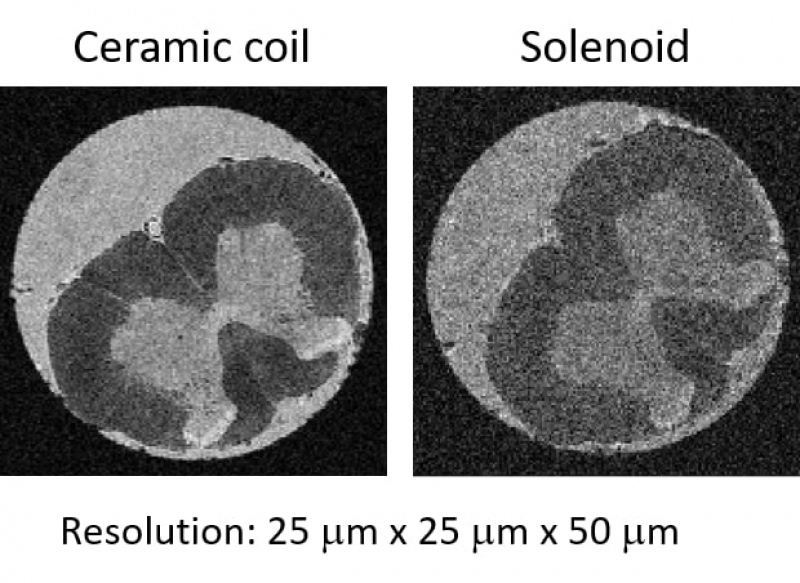
“Many ceramic materials with a high epsilon have a drawback: their permittivity greatly depends on their temperature. For example, a deviation by five degrees from room temperature leads to a complete detuning of the resonance frequency of the probe in the tomograph. But it turned out that this property can be used for our benefit: the coil and the sample were put into an electrically controlled thermostabilization device that is used for controlling the temperature of living objects. With the help of this device, we succeeded in attaining the necessary temperature of the resonator that allowed us to adjust the coil’s frequency and sustain it throughout the whole scanning procedure. This way, we can check whether our coil is properly adjusted by electronic means, and tune it remotely without having to extract the sample,” explains the scientist.
Application prospects and future research
In magnetic resonance microscopy, the resolution of images depends on the signal-to-noise ratio that can be attained during the particular time of data collection (the more time you spend, the better ratio and hence the better image quality you can attain). Still, in the case of most biological samples, for example living cells and extracted tissue, the scanning has to be conducted very quickly, before the sample gets spoiled, explains Luisa Ciobanu.
“The twofold increase in sensitivity that we obtained thanks to the ceramics-based probe will quicken the collection of data by four times at the same level of resolution. Therefore, such a device is promising for biological research. Apart from magnetic resonance microscopy, the probe can also be used in NMR spectroscopy (nuclear magnetic resonance spectroscopy – Ed.) which is nondestructive as opposed to mass spectroscopy. But due to lower sensitivity, NMR spectroscopy requires more amount of a sample. The ceramic probe that we described in our article can offer many applications like those associated with the identification of new natural products, analysis of metabolites or the study of the deterioration of agrochemicals and pesticides,” says the researcher.
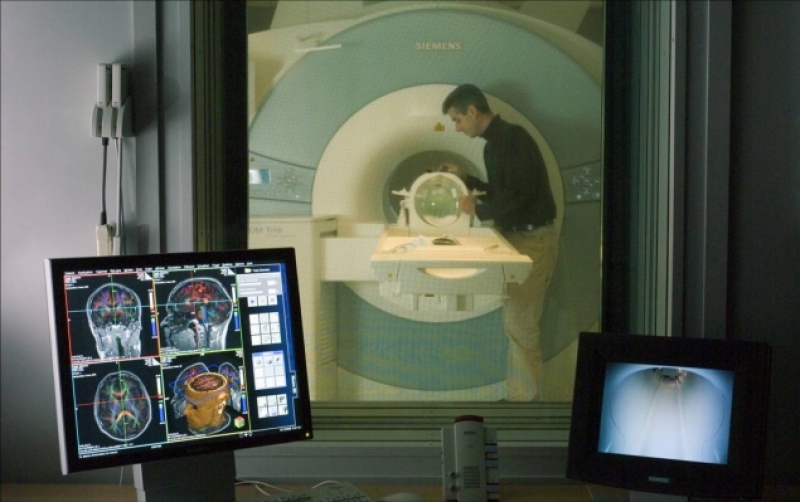
Specialists of Ceramics Jsc. have already started the development of a new set of materials in order to produce an even more sensitive probe for 17T tomographs. According to Stanislav Glybovski, the researchers are also interested in creating probes of other form and size for use with other types of biological samples and in vivo brain study of laboratory animals.