Nobel Prize in Physics
According to Alfred Nobel's will, the Nobel Prize in Physics shall be awarded to the person who made the most important discovery or invention in the field. The first to receive the award was Wilhelm Röntgen for his discovery of X-rays in 1901. To date, the total list of recipients has more than 200 individuals.
The award is often given for fundamental research, the possible applications of which may not be immediately obvious. Let’s find out which discoveries received the prize recently and how they changed the world.
Attosecond pulses of light. In 2023, the Nobel Prize was awarded for “experimental methods that generate attosecond pulses of light.”
An attosecond is a unit of time equal to one billionth of one billionth of a second (1×10-18 of a second). It is so short that the heart, which beats approximately once per second, is 18 orders of magnitude – or a billion times billion – longer than an attosecond. At the same time, the universe is about 13.8 billion years old, which is 18 orders of magnitude greater than the speed of a heartbeat. In other words, one second is the age of the universe if compared to an attosecond.
Although the research started as purely fundamental, the scientists are now looking for ways to implement attosecond pulses. For one, they can be useful in disease diagnostics as molecules react differently to short laser pulses; the same properties can be employed to produce molecular imprints to distinguish cells and molecules for biology and biomedicine needs.
Read also:
Nobel Prize in Physics 2023 Awarded to Researchers Who Made the Impossible Possible
Neutrino oscillations. Another fundamental study was honored by the Nobel Prize in 2015; the award was given “for the discovery of neutrino oscillations, which shows that neutrinos have mass.”
The neutrino is a tiny electrically neutral particle that does not have mass according to the Standard Model of particle physics (the theory describing the basic components that make up the universe and how it was formed – Ed.). The fact that the neutrino does have mass shows that the Standard Model needs to be supplemented, collider physics – to be further explored, new telescopes – to be launched, and cosmic rays – to be studied.
Every second, billions of neutrinos pour through us, but we can barely notice them. Teenagers may describe their lives similarly. They think the world is cruel and unfair, people do not pay attention to them, and family and teachers cannot understand them. That is much like neutrinos: billions of these particles are now passing through us, and they do not care about us at all.
However, sometimes neutrinos collide with other particles, causing different reactions and producing new charged particles. The radiation of such new particles is detected several times per year by Cherenkov detectors. These particle detectors can indirectly determine the mass of particles or separate lighter particles from heavier ones. Such detectors can be found in only a few places on the planet: neutrino detector Super-Kamiokande (Japan), IceCube Neutrino Observatory (the South Pole), Baikal Deep Underwater Neutrino Telescope (Lake Baikal, Russia), and some others.
During a neutrino’s interaction with other particles, three types of particle pairs appear: electron, muon, and tau. Each of the three neutrinos is in a superposition, meaning they have three different masses and therefore travel at different group velocities. As they travel through space, they transform – or oscillate – from one type to another. The scientists’ discovery of neutrino oscillations, which proves that neutrinos have mass, brought them the Nobel Prize in Physics. As for applications, their study sheds light on processes occurring inside the Sun and other stars, and helps remotely monitor the state of nuclear reactors to prevent accidents.
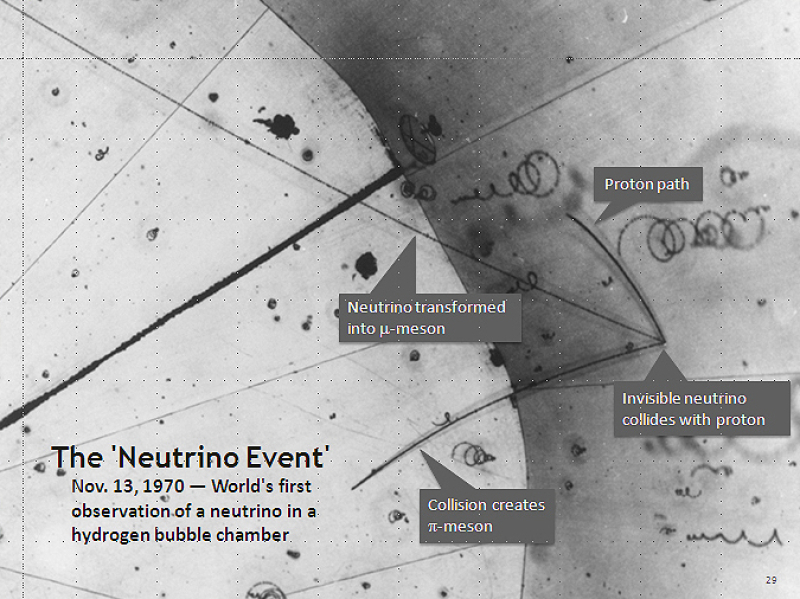
One of the first observations of a neutrino in a bubble chamber. Credit: Argonne National Laboratory / wikimedia.org (public domain)
Higgs boson. The 2013 Nobel Prize in Physics was given "for the theoretical discovery of a mechanism that contributes to our understanding of the origin of mass of subatomic particles.” The Higgs boson was discovered by researchers at the Large Hadron Collider (LHC) located at the European Organization for Nuclear Research (CERN). To visualize the collider, you can imagine St. Petersburg’s Gazprom Arena – but with a circumference of 27 km.
The LHC can accelerate particles and crash them into one another at the speed of 6.5 TeV; several detectors capture the particles produced by the impact. Every year, the collider generates petabytes of data that cannot be processed manually. Therefore, these days physicists opt for AI in addition to specialized software to process data and identify unusual patterns such as neutrino oscillations. The discovery of the Higgs boson heralded a new age of physics; it helped researchers understand that physics in the 20th century was headed in the right direction and mathematical models produced initially for elegance and aesthetic purposes were in fact describing reality.
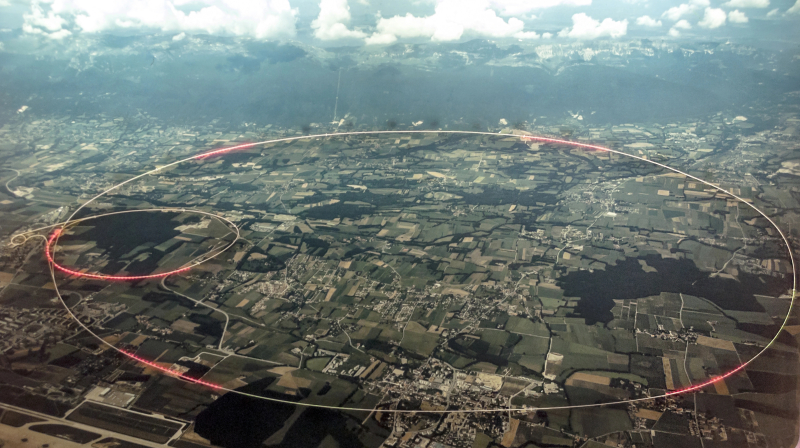
CERN. Credit: GranTotufo / photogenica.ru
Applications
Data transfer. Many modern technologies owe their existence directly or indirectly to accelerator physics. For instance, scientists at CERN had to transfer massive sets of data generated by the accelerator and so a special protocol was produced for the purpose; it is now used to transmit data via the internet.
Transport. The same is true for superconducting magnets. Originally created for particle accelerators, the technology turned out to be beneficial for Maglev trains that can now be found in China and Japan.
Medicine. Accelerator physics found many uses in medicine, too: X-ray imaging lets us see what is happening inside the body in real-time and proton therapy targets cancer more precisely, without harming healthy tissues, as well as reduces the risk of side effects and cancer recurrence as opposed to X-rays beams
Art. Accelerator physics benefits art, as well. In 2008, researchers discovered a previously unknown portrait of a woman beneath the paint of Vincent van Gogh's Patch of Grass using fluorescence spectroscopy and synchrotron radiation.
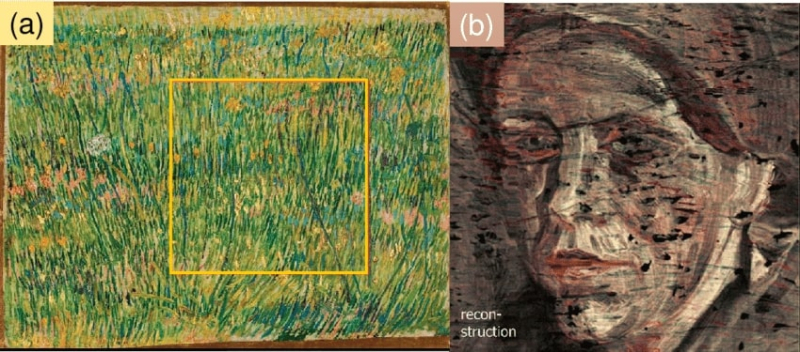
(a) Photograph of Patch of Grass by Vincent van Gogh; (b) Tri-tonal reconstruction. Credit: Koen Janssens / ResearchGate
Next year, the Siberian Ring Photon Source (SKIF) will be launched in Akademgorodok, Novosibirsk. SKIF is a synchrotron radiation facility for applied problems of physics, biology, medicine, chemistry, catalysis, and energy of the future, which can be utilized to analyze and create new composite materials, vaccines, antibacterial medications, as well as materials for hydrogen, thermonuclear, and other types of alternative energy sources.
The prospects
In terms of technology
The latest Nobel Prize in Physics was awarded “for foundational discoveries and inventions that enable machine learning with artificial neural networks.” The decision divided the community of physicists into two camps: the first group was bothered by the fact that the award was given for something that is not physics; and the second one welcomed the further use of AI in physics research.
But the prime task on the agenda is to figure out what AI has become for us: is itThe Iron Nanny from Russian cartoon KikoRiki, something from The Matrix Universe, or the Terminator.
Any equipment, telescopes, and accelerators generate huge amounts of data that needs to be processed. What was first conducted manually was then simplified by the use of specialized software and is now handled by AI. Although the technological shift is a rather natural next step, the use of AI may generate fake events in physical experiments that scientists will have a hard time distinguishing from the real ones.
Read also:
Nobel Prize in Physics Awarded for Discoveries in Machine Learning and Neural Networks
In terms of philosophy
In the early 20th century, there was a philosophical paradigm behind contemporary science that explained how scholars receive awards and make discoveries. It went as follows: work long and hard, make a breakthrough, and receive an award for your work. The same paradigm is still taught at schools and universities. But the reality is that the modernist era is over – and with it the dreams of the completed Standard Model.
In today’s world, scientists can work on a problem for decades and still not get a result. This happened to the Tevatron collider, which almost discovered the Higgs boson but did not have enough energy to make the breakthrough – which was then accomplished by the LHC.
Nevertheless, it is important to realize the LHC may not discover any new particles, dark matter, or any other physical phenomena in the near future.
Also, we need to be ready to hear that we will not be able to achieve the needed levels of energy and time in the coming decades.
Take the Grand Unified Theory for example. This theory explains what happened in the first five minutes after the Big Bang and how the fundamental forces of nature were split into strong, weak, and electromagnetic interaction.
To recreate such conditions, we would require energy of 10-16 GeV, whereas the LHC can do as much as 10-4 GeV, which is 12 orders of magnitude (i.e. a trillion times) less. To confirm a more global theory of everything, which combines the three fundamental forces and gravity, scientists need even more energy – the so-called Planck length (1.6x10-35 m) and Planck time (10−44 seconds). Some may recall that only in 2023 the Nobel Prize was awarded for generating attosecond pulses of light, which are only equal to 10-18 seconds.
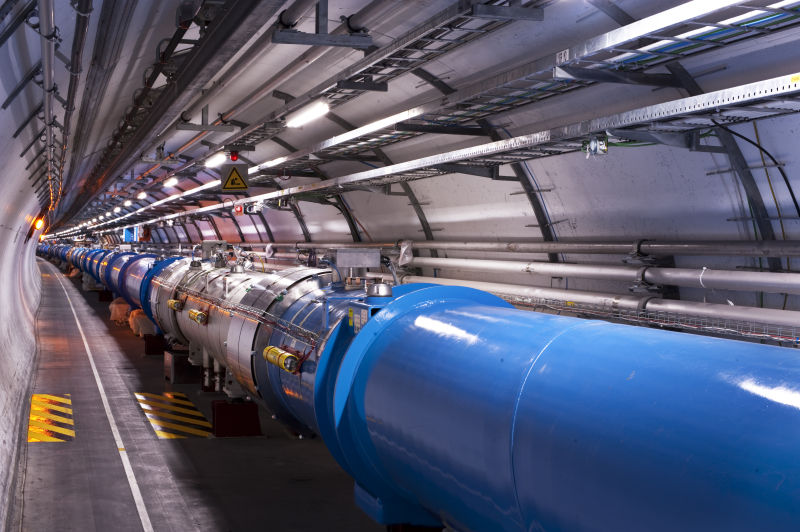
Large Hadron Collider (LHC). Credit: By Maximilien Brice (CERN) - CERN Document Server / wikimedia.org (CC BY-SA 3.0)
Does it mean that we should stop our studies? No, even ongoing work on fundamental scientific problems helps develop different applied technologies that can benefit society. And it may be that we will see more discoveries in these fields that will bring us closer to finding new particles and their interactions.
Dmitry Karlovets’s lecture was part of the pop-sci festival SciFest by ITMO’s School of Physics and Engineering during which scientists spoke about their research at the school and answered applicants’ questions on admissions and education, starting a career, and current trends on the labor market.