The Microwave Theory and Techniques Society (МТТ-S) is the largest international society of scientists conducting research in the fields of radiofrequency, microwave, and THz radiation. The organization is part of the Institute of Electrical and Electronics Engineers and regularly holds fellowship contests for students.
The IEEE MTT-S Graduate Fellowship can be awarded to Master’s and PhD students who work on the most promising projects in their field. Those wishing to take part in the contest can apply in one of two categories: General Category and Medical Application. The award itself amounts to $6,000. Annually, eight physicists studying microwave electronics and engineering receive the fellowship in the general category, while only two scientists are awarded in the Medical Application category.
Over the years, different ITMO University researchers won the award in General Category, including Pavel Belov, dean of the Faculty of Physics and Engineering, Alexey Slobozhanyuk (in 2016), senior researcher at the faculty, and Dmitry Zhirihin (in 2020), one of the faculty’s PhD students.
It was Anna Mikhailovskaya, however, who became the first Russian young scientist to be awarded in the Medical Application category. We talked to her about her research interests, the results of her work, and plans for the future.
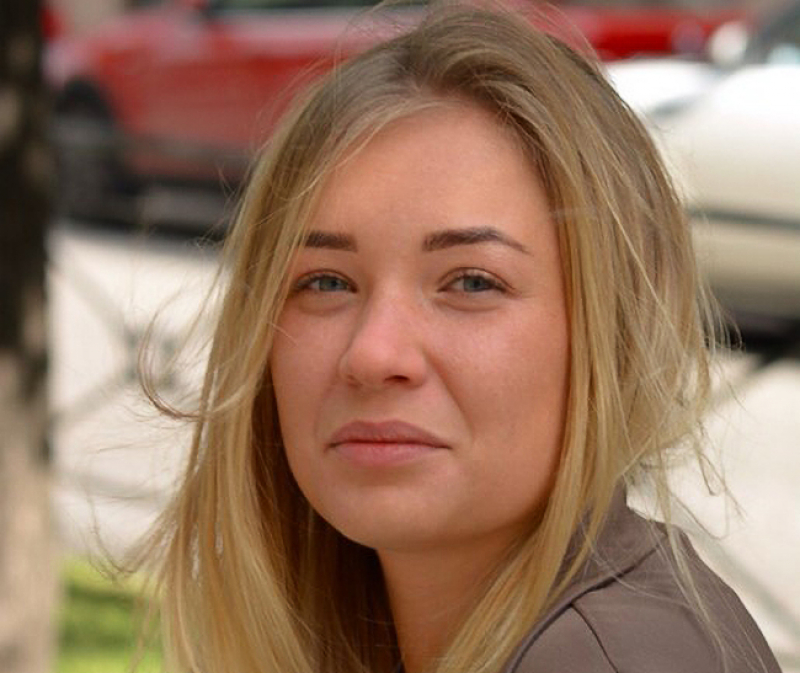
No one has done it yet
Why did you decide to apply for the fellowship?
It’s a famous contest that offers many opportunities for young scientists. It’s well-known among physicists working in the field of radio- and antenna technology. At the same time, the application process is not that complicated – you just have to describe your project and explain what you are going to do and how, as well as give an overview of what you have already done in this field.
When I applied, I didn’t think I would win – the competition is much higher in the Medical Applications category. But I decided to try and it was worth it, I won.
What was the project you applied with?
In my work, I develop radiofrequency coils for MRI, which is currently the most promising tool for medical visualization thanks to its information richness and data precision. At the same time, MRI is a non-invasive and thus a relatively safe method of diagnostics.
For instance, MRI gives us a unique chance to study the metabolic and microvascular functions of skeletal muscles by means of measurement of phosphorus and hydrogen protons. That’s why we need coils for lower limbs that work on two nuclei – one on phosphorus and one on hydrogen. In my project, I plan to design and make prototypes of wireless two-core radiofrequency coils for clinical MRI systems that will significantly increase the signal-to-noise ratio and image resolution for the same magnetic field, as well as bring down the scanning time with no loss in the image quality. There are currently no such coils in Russia or anywhere else in the world, which challenges diagnostics and thus makes it harder for radiologists to prescribe treatment.
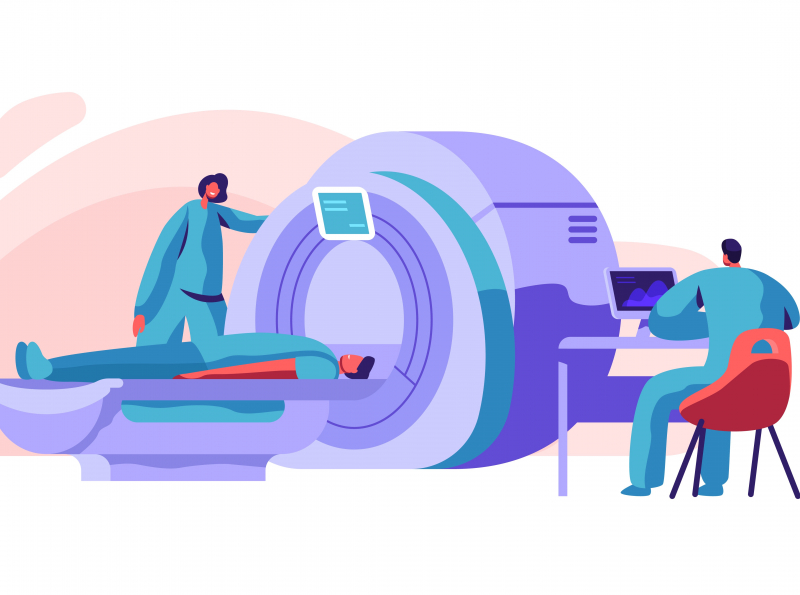
How can this project increase image quality?
MRI is based on exciting magnetic resonance in the human body and registering its radiofrequency feedback. The excitation happens by creating a uniformly spread radiofrequency magnetic field in the scanner. At the same time, a strong constant magnetic field has to be created inside the scanner as well.
Clinical scanners usually have a relatively low constant magnetic field – around 1,5-3 Tesla, which is enough for most diagnostic procedures. It is the combination of a radiofrequency magnetic field and a constant magnetic field that affects hydrogen atoms in body tissues. In these conditions, nuclear magnetic resonance is possible, which leads to a synchronous strong change in the direction of nuclear spins – protons. As the excitation ends, atoms steadily return to their initial states. However, during this transition protons emit radiofrequency radiation. Different organs and tissues will produce different radiation due to their varying proton concentration, which allows to contrast them in an image. This contrast is registered by a special antenna, the signal from which is then sent to receivers, digitized, and analyzed by a computer. Historically, antennas in MRI scanners are referred to as radiofrequency coils.
In general, we can increase image quality in three ways: by improving computer data processing; increasing the constant magnetic field, i.e. placing a bigger magnet inside the scanner (but the consequences it might have on the human body are not fully known); or by working with radiofrequency coils. And this latter is my field of work – one can say I deal with the hardware.
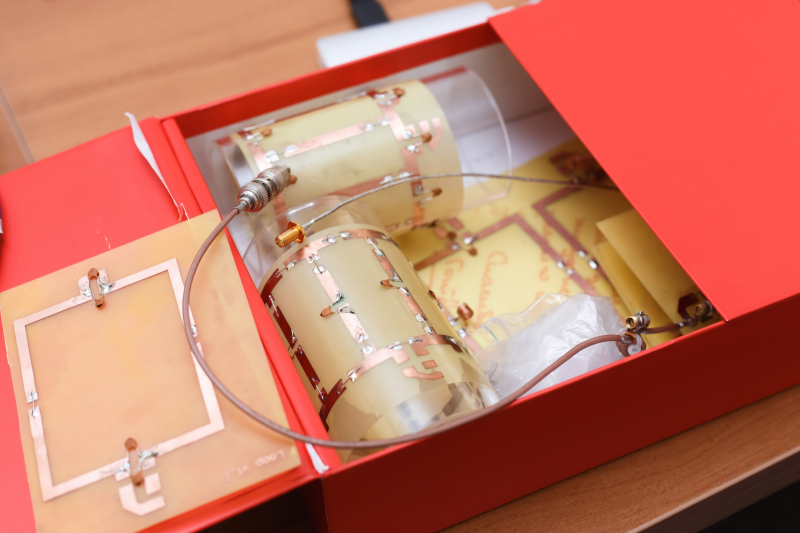
Why do we need this?
The better the MR image, the higher the chances of identifying pathologies in patients – which means early diagnostics and prevention of unwanted consequences. For instance, with MRI, to spot early coronary heart disease in sportsmen doctors need to monitor creatine phosphate regeneration in skeletal muscle. To do this, we need to create a coil that would allow scanning on two cores (in this case, phosphorus and hydrogen) in clinical MRI scanners.
There is no such thing in the world yet. Most of the double-frequency coils are made for research scanners that work with very high electromagnetic fields.
As far as I know, your application was based on your earlier projects. Can you tell us more about it?
Together with my colleagues at the faculty, I have acquired substantial experience in developing radiofrequency coils. Our research group explores the ways to use metamaterials, metasurfaces, and special ceramics as new and more efficient send-and-receive antennas for MRI. We study super high-quality, ultrabroadband, time-varying, and practically invisible devices to see if we can apply them to expand the capabilities of MRI scanning.
I sent my application in November – by that time I had a general understanding of the design concept, as well as preliminary models of the hybrid coil. There is a lot left to do, but I think that in a month and a half we will be able to start assembling the coil and testing it in the anechoic chamber. After that, we will move on to clinical trials in an actual scanner: first on phantoms (objects that imitate the parameters of the human body) and then on volunteers.
Experimenting is fun
How did you get into this particular field?
I have been developing radiofrequency coils since 2017. As I was finishing my Bachelor’s at ITMO, I understood that I wanted to do science. At the same time, it was important for me to work with something feasible that can help people and not with abstract research.
My parents are doctors, so I was interested to find something in medicine. In 2017, this field at the faculty of physics and engineering was developed by a group headed by Pavel Belov. I got on the team and into the Master’s program Physics of Radiofrequency Technology, which provides training in MRI and antenna devices.
What do you do for the project? Is it only preliminary calculations, or do you also conduct experiments and solder wires?
I do everything, that’s how our faculty works. We start with a literature review (so as not to reinvent the wheel) and then do preliminary modeling to see if our design works. After that, we move on to more precise calculations with a voxel model of the human body and a model of an actual scanner to check the signal-to-noise ratio and radiofrequency safety. Next, we move on to assembling radiofrequency coils. We have a great laboratory, there are many engineers that are ready to help. Although, if I remember correctly, we have always assembled the coils ourselves in our team. Then, we run experiments in the anechoic chamber and, finally, on actual clinical scanners. As an outcome of this work, we write a scientific paper.
Which part of the work do you like most?
I would say modeling – you can obtain interesting results at this step. But most of all I like experiments. They are cool and fun, and we have a great team that can help you with every step. Experiments are the final stage, this is where you wait for your results to be proven.
I remember one time when we were running an experiment with a volunteer in an MRI scanner. They had to spend around three hours inside. At one time, we started getting images with movement artefacts – it turned out that our volunteer fell asleep and started moving, thus messing with our data.
There are also things I am yet to like, such as writing papers. I am not big on writing, so i am grateful to have my supervisor and my colleagues, who are always ready to help with that.
What are you most inspired by? What do you strive for?
My biggest inspiration is seeing the result of my work, being able to touch it, and knowing that someone can benefit from it. It’s important to realize that you are not doing that just to get it published or earn money – you are doing research so that it helps people.
Are you planning to continue working in academia after getting your PhD or will you consider a career in the industry?
I am currently doing a double degree PhD program and spend most of my time in Israel, at Tel Aviv University. Next, I am planning to remain in academia and search for a postdoc position – in Russia or somewhere else. I have three more years of PhD studies ahead of me, so I still have time to think about my next move.
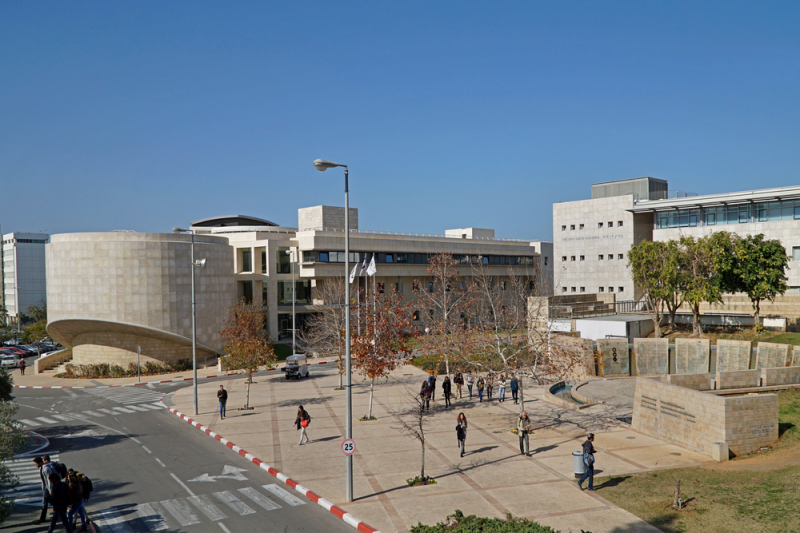
And what about the topic – will you work in the same field or find something new?
Actually, my topic has already changed a little. I still work with MRI, because I love this field, but my main focus is currently radio-frequency identification (RFID) and multiple-input and multiple-output (MIMO) technologies.