As a spintronics expert, could you educate us more on the field?
To be brief, spintronics is a field of quantum electronics that studies spins, that is the intrinsic angular momentum of a particle, in solid-state devices. Spintronics fundamentally differs from traditional electronics: here, spin rather than electric current is used to transfer information or energy. With the advances of spintronics, researchers can achieve higher data density and its transmission speed in logic devices.
How did you get into spintronics and what made you become a scientist?
About to receive my university degree, I realized that I didn’t want to stop studying: learning something new was essential for me. As a final-year student, I was fortunate to get a position at a laboratory. So, when an opportunity to do my PhD arose, I took it. What else brought me to science was, perhaps, my curiosity about the world that manifested itself not only in science but also in nature observation and some other fields. Sometimes I wish I had several lifes to experience it all. I settled on spintronics because I find it inwardly beautiful. The way I see it, scientific endeavors are somehow similar to investigations when you want to get to the bottom of things.
What projects have you worked on?
My career as a scientist began at Immanuel Kant Baltic Federal University in Kaliningrad where I thoroughly studied composite multiferroics in thin-film forms. These are special-use structures, tens or hundreds nanometer thick, that exhibit magnetic and ferroelectric phases (layers) and make it possible to convert an external magnetic field into electricity. Such materials are used in a range of sensors, including those in cars, phones, or medical devices, as well as power supplies.
Then, in my PhD studies, I switched to a different type of composite object, namely glass-coated amorphous ferromagnetic microwires. Because of their amorphous structure and internal mechanical stresses, these materials display exceptional magnetic properties. Although I mainly conducted experiments, in recent years, I got to try my hand at theoretical modeling, as well.
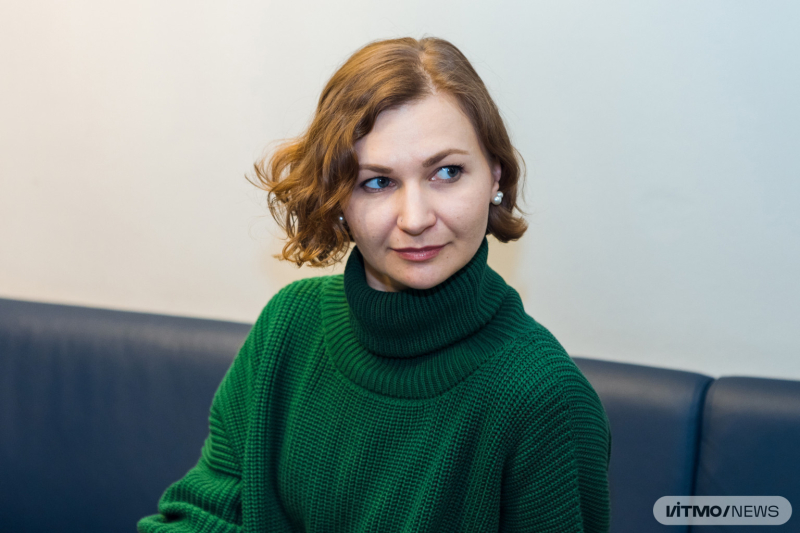
Ksenia Chichay. Photo by Dmitry Grigoryev / ITMO.NEWS
Why did you decide to carry on with theoretical studies?
In some cases, theoretical modeling offers a more detailed close-up look at some processes that occur within objects. Studying microwires, I wanted to have a peek inside them and visualize their micromagnetic structure. While thin films can be easily studied via microscopes, microwires are harder to investigate due to their cylindrical shape and insufficient space. This was my first push to give theoretical modeling a try.
In 2015, I went to Tohoku, Japan, to work with skyrmions, magnetic vortices that can behave as particles. This was the first time I was able to see inside an object and study its configurations via simulations.
It was also my first time being a part of a theoretical team. Thanks to this experience, I learned that theorists look at things differently. While experimentalists measure characteristics they have on hand, theorists are able to see an object the way it might be.
Starting 2019, I’ve been closely working with Forschungszentrum Jülich. Together with the center’s researchers, we’re investigating both vortices and more complex structures, like multi-layer skyrmions, as well as their dynamics. That was when I made a choice to pursue theory and modeling. I’m confident that my versatile experience shaped me as a researcher who incorporates multiple perspectives into their studies.
How did you learn about ITMO? And what made you decide to join the ITMO Fellowship program?
I learned about ITMO a few years back when I participated at PI School by SCAMT Institute. Then, I thought ITMO was a rather modern university with active science, promising developments, and highly-qualified research teams. Last year, when I was looking for a new job, I thought of professor Valery Uzdin. I knew that his research team dealt with topological solitons. I was interested in the topic so I reached out, and he told me about the program.
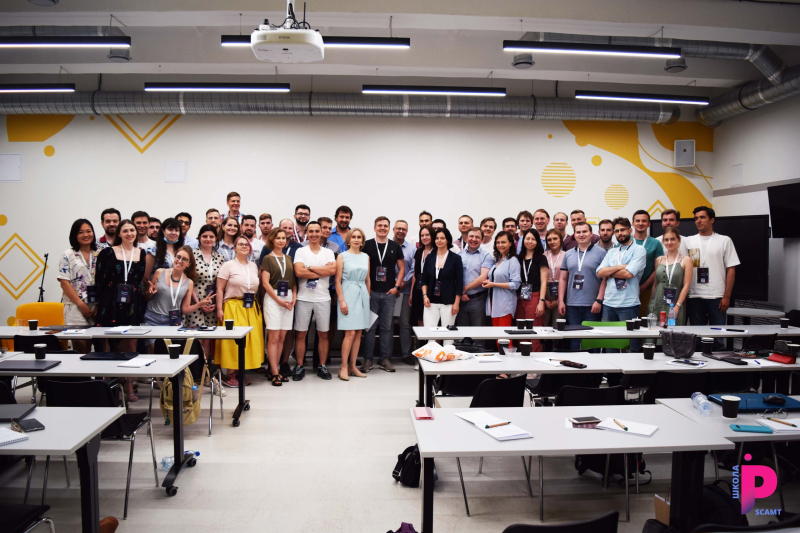
PI School by SCAMT. Credit: scamt.ifmo.ru
What are your thoughts on the program? How different is it from other programs?
I interned a lot both in Russia and abroad. In my opinion, ITMO Fellowship, unlike other Russian programs, has more in common with those by universities abroad: the application form is rather brief and concise and the application process overall doesn't come with a massive amount of paperwork. I love that applicants are assessed regarding not only their competencies and their compliance with the interests of the research group but also skills and knowledge they can enrich their future group with. This is a valuable approach.
What are you working on at ITMO? What goals are you working towards?
At ITMO, I study magnetic topological solitons – localized imperfections and defects representing special states of matter, such as magnetic vortices. Other examples of such phenomena include skyrmions and domain walls, which are interfaces that separate different areas of uniform magnetization.
What are we working towards? Well, right now there is a trend towards miniaturization in science: in order to store more data, we need to make our data storage devices smaller. Unfortunately, there is a limit to how small we can make them, since at a certain size the device becomes vulnerable to outside interferences, such as temperature changes. That’s when topological defects can become useful, since they each have a certain energy threshold one needs to surpass in order to destroy them. Our research group studies how various outside influences affect the stability of such objects.
I also research how these objects move through amorphous ferromagnetic cables, which was something I worked on when studying for my PhD. I have a wealth of experimental data, of which one part is already explained while another remains unpacked as of yet. In order to analyze it properly, we need to look at the inside of these objects. That’s why I use modeling to study how domain walls move and change within such structures. My team and I even created a special software module that allows us to represent these objects in more detail.
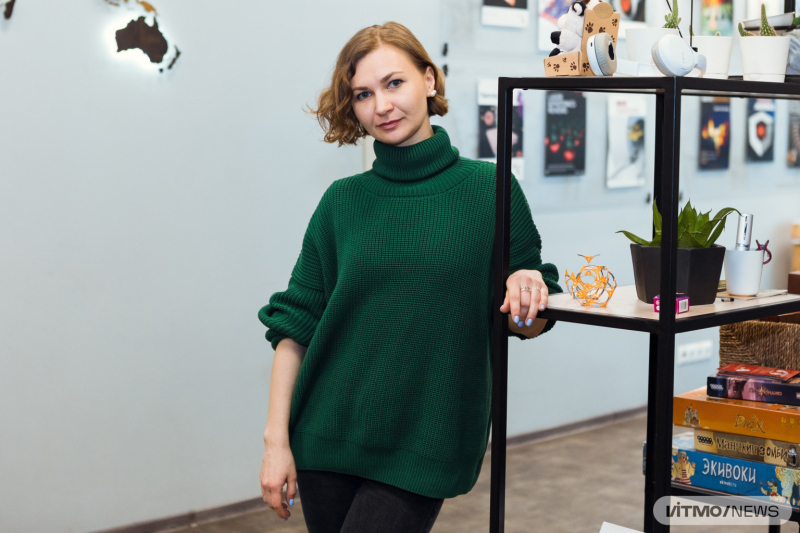
Ksenia Chichay. Photo by Dmitry Grigoryev / ITMO.NEWS
What are the practical applications of your research?
The results of our work could be used to create new methods of recording, storing, and transferring data.
For instance, within any magnetic material there are domains – areas with uniform magnetization (that means that the individual magnetic moments of each atom within that area are all aligned to point in the same direction). A nano- or micro-sized cable made from a magnetic material can be divided lengthwise along the border of each domain, producing pieces with opposite magnetic moments. When placed inside a magnetic or an electric field, domain walls can transfer information by moving along the cable.
In 2008, physicist Stuart Parkin proposed using these properties to create a new type of information storage: racetrack memory. It has the benefit of not needing to move neither the recorder nor the sensor, relying solely on the movement of the domain walls within the cable. That means racetrack memory devices have no moving parts, which eliminates the risk of a breakdown that is present when using traditional recorders. Recording rate will depend on how quickly domain walls can move, so we currently research ways to speed up their motion.
There are also skyrmions to consider, as they can also be forced to move with much less dense electric currents. We aim to study whether this technology can be used for long-term data storage. One possible application is in logical devices which process digital data (e.g. processors within smartphones and computers). This requires us to experiment with topological solitons in realistic conditions, such as non-uniform magnetic fields containing defects. These imperfections and temperature fluctuations are crucial when it comes to the stability of the structures that we study.
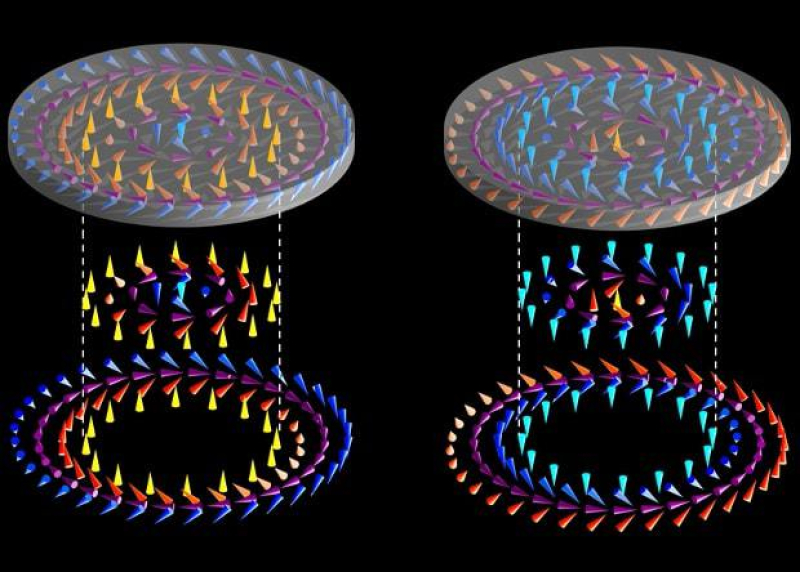
A spin structure of skyrmions-targets with two different vortices. Credit: Physical Review Letters, 2017
You focus primarily on theoretical research. Unfortunately, not everyone sees the value in studying something with no immediate practical application. What would you say to these people in order to make them appreciate fundamental science?
Applied research usually only focuses on studying something that will be useful here and now, or at least in the near future. Meanwhile, in fundamental science you can discover something that has no obvious value in the present, but will become immensely useful in the future. For example, multiferroics (another former research subject of mine) exhibit a magnetoelectric effect which was predicted way back at the end of 19th century. This effect was then first observed in the 1960s, but it had no practical application because of its small size. But a couple of decades ago scientists came up with a way to replicate this effect in complex composites, which offer the perfect conditions for its use.
Skyrmions are another example, as they were first proposed as a concept by physicist Tony Skyrme in the 1960s. Their existence was then predicted by Alexey Bogdanov and Dmitry Yablonsky 30 years ago, but it was just 10 years ago when their existence was actually proven during an experiment.
What do you like to do outside of work?
I participate in solo and partner dance competitions in hustle, discofox, and west coast swing. What I adore the most about dances is improvisation and freestyle when you can express yourself freely through music and movement beyond a specific dance genre. It seems that the same desire can be traced in my academic career. After all, it’s at the intersection of different fields, whether it’s dances or science, is where the true magic happens.