Today, a great deal of scientific discoveries have to do with nanoparticles. Among other things, they help scientists detect impurities in gasses and liquids as well as purify medication; they can also be useful in bio-visualizing tumors and treating them through phototherapy. But in order to create new nanoparticles with new unique properties, we must first study their qualities and their interactions with their surroundings.
“If you were to take a kilogram and a half-kilogram of gold, in our layman’s world they’d only differ in mass and size, but still possess the same basic properties. In the nanoworld, it’s all different: the properties of a gold nanoparticle are dependent on not just its chemical makeup, but its shape and size, too. The latter affect the qualities of all nanoparticles and therefore their efficacy in all applications – from cancer treatment and solar power to quantum computing. That’s why it’s important to know how to create nanoparticles of the right shape and size and how to check that you’ve done it just the way you needed to,” explains Dmitriy Shcherbinin, one of the authors of the study and a senior researcher at the laboratory Nonlinear Optics of Condensed Media at the International Research and Educational Center for Physics of Nanostructures, ITMO University.
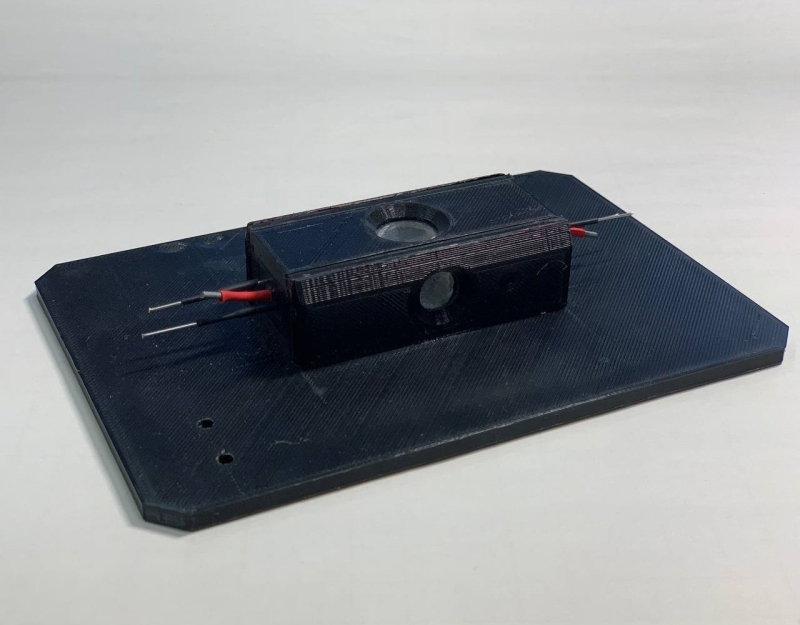
The electrodynamic trap. Photo courtesy of Maksim Semynin
Different types of microscopes and spectrophotometers allow scientists to examine the physical (size, shape, and charge) and optical (emission, absorption, and scattering spectra) properties of whole ensembles, which are massive amounts of particles. But what if it’s just one? Researchers will sometimes produce samples in which the particles are far from one another, then find the individual particles with an electronic microscope and examine the shapes and sizes. Then, they’ll switch to a fluorescence microscope and find the same particles to measure their optical properties. This method is taxing on both time and effort.
Another option is to use optical tweezers. This device, quite similar to an optical microscope, can fix a singular particle in place and conduct its structural and optical analysis. This approach, however, requires in-depth knowledge of optics, a lot of time and effort, and on top of that limits the potential for upscaling of such analysis. First, not all nano-scale objects can be grabbed with optical tweezers. Second, the device is always a unique set-up that must be fine-tuned to each occasion.
Researchers from ITMO’s Center for Physics of Nanostructures have designed a new tool for the study of singular nanoparticles – a manipulator add-on based on the electrodynamic trap of their own design. The device forms an electrical field that causes particles to levitate and thus holds them in a specific spot.
“What are the advantages of an electrodynamic trap compared to optical tweezers? In the case of tweezers, you need to design a specific and quite unwieldy optical framework for each particular task. So, moving the set-up into another lab becomes a non-trivial task. Using the electrodynamic trap technology, we’ve developed a portable manipulator add-on that can “catch” an individual particle. It works with any commercially available optical microscope, it doesn’t require any special knowledge to be used, and it can help study particles of every shape in an aerial environment, which doesn’t affect the properties of the object,” explains Vadim Rybin, one of the authors of the study and an engineer at the laboratory Nonlinear Optics of Condensed Media at the International Research and Educational Center for Physics of Nanostructures, ITMO University.
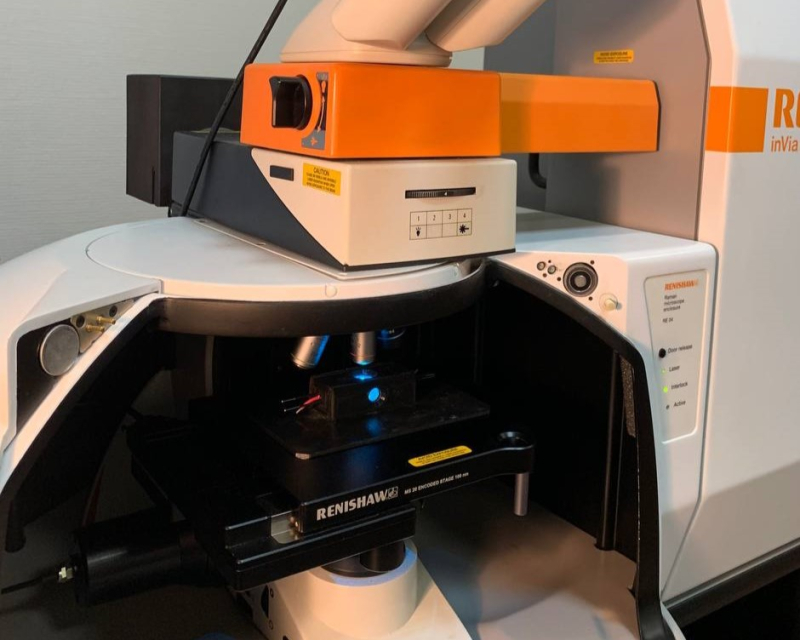
The device in use, inserted into a microscope. Photo courtesy of Maksim Semynin
The electrodynamic manipulator resembles a small, hermetically sealed rectangular box that can be inserted into the object table of a microscope. One of the walls of the box contains an opening through which the particles can be inserted via syringe. Inside the box are six electrodes – four long, two short. They all serve as “electric shepherds” and the particles – “electric sheep.” Using a specific amplitude and frequency of electric current, the electrodes attract particles of the necessary shape, size, and mass and, owing to the resulting electric field, keep them in place. Thus, it’s possible to work with a selection of particles of varying mass and charge. At the same time, the optical properties of a particle aren’t important and don’t affect its ability to stay fixed by the electric field. As a result, the “shepherd” always grabs the right “sheep” and the scientists know where to point the lens. The other particles will remain in the trap and won’t impede the analysis.
“The ability of an electric field to keep the particle in place allows us to know in advance where the particle is going to go and, in just a few moments, move the lens there. With the usual methods, you’re forced to spend time looking for the right object. With ours, you can examine any charged objects of a size between dozens of nanometers to hundreds of micrometers. The area of application isn’t limited, either – from biological structures, for use in early diagnostics of, say, cancer, to the synthesis of new materials for nanoengineering or the study of particles for fundamental physics research,” says Semyon Rudyi, one of the authors of the study and the head of a laboratory at the International Research and Educational Center for Physics of Nanostructures, ITMO University.
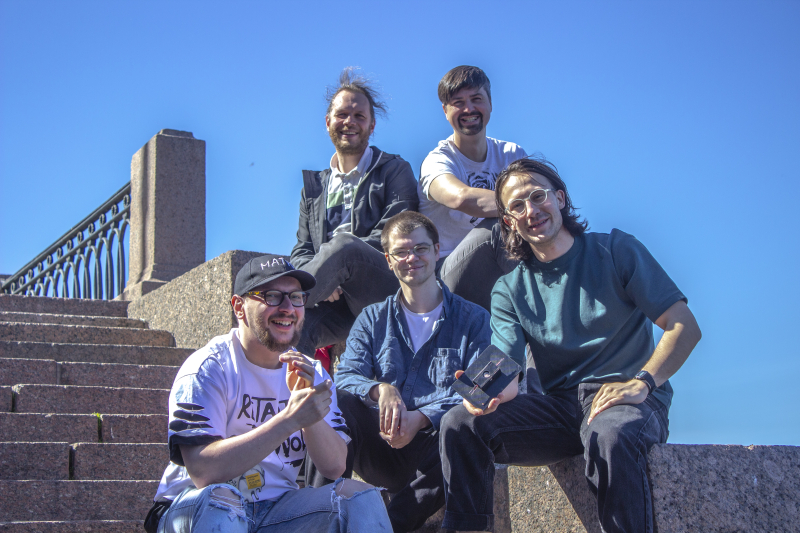
The creators of the device. Photo courtesy of Darina Klimenko
In the future, electrodynamic traps may be combined with optical traps to produce a hybrid mechanism for capture of multiple particles. With such an instrument, it would be possible to manipulate two different nanoparticles and cause them to interact. This functionality would be helpful in screening of biological tissue and identification of diseases, such as when studying the rigidity of cell membranes or artificially inseminating fruit flies for genetic research.
The research project is supported by grant No. 24-22-20042 of the Russian Science Foundation.