Chirality is a fundamental symmetry property that appears in molecules, nanoparticles, and macro-objects. An object is chiral if it is distinguishable from its mirror image and cannot be superimposed onto it when rotated or moved. Chiral objects can rotate the plane of linearly polarized light or absorb circularly polarized light depending on its direction.
Although physicists have known about the property since the 19th century and applied it in molecular systems, the field has taken a step forward with the advent of technologies that enable scientists to synthesize nanostructures with distinctive optical properties, such as plasmonic nanoparticles, the optical chirality of which manifests itself through geometrical asymmetry.
Plasmonic nanoparticles are nanostructures made of noble metals, primarily gold or silver, that can localize electromagnetic fields at scales smaller than an optical wavelength. The exposure of metal particles to a resonant wavelength stimulates collective oscillations of conduction electrons (plasmons) on the surface. Such oscillations are particularly responsive to external conditions and change their optical response – in other words, their own frequency – depending on these conditions.
This property finds use, for instance, in biosensing, where it is utilized when detecting biological samples, identifying impurities and ions of heavy metals, purifying medications, and so on. Alternatively, if the geometry or composition of plasmonic nanostructures is modified, the nanostructures obtain chiral properties that are superior to those of molecular systems.
Due to this, the properties of chiral plasmonic nanoparticles result in more accurate optical responses, allowing specialists to analyze and detect substances according to multiple parameters at once, such as plasmon frequency or ellipticity. Chiral nanoparticles possess huge potential in various applications, like molecular probing or the separation of chiral molecules into specific enantiomers; they can also serve as passive optical polarizing elements.
Although chiral nanoparticles are generally produced with chemical methods (when particles are grown from other molecules) or electron-beam lithography (a practice of applying particles onto a polymer surface via an electron beam), the problem is the former method is hard to conduct, whilst the latter is highly expensive.
The solution
Researchers from ITMO’s International Research and Educational Center for Physics of Nanostructures devised a novel method of producing plasmonic nanoparticles with chiral properties. Per the method, gold nanoparticles are placed within a silicate matrix, where they are thermally processed to form two- and three-dimensional nanoparticles. After that, they are irradiated with a circularly polarized beam in the visible range. Depending on whether the electromagnetic wave “twists” clockwise or counterclockwise, specialists remove molecules that absorb the light of that frequency, leaving those that resonate. The result is an ensemble of nanospheres of various diameters, with chiral properties defined by the “twist.”
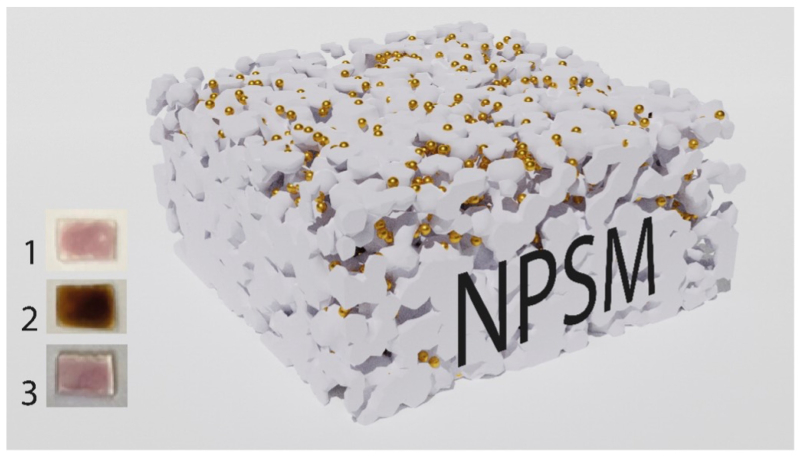
A 3D model of the composite structure with a racemic ensemble of gold nanoparticles embedded into a nanoporous silicate matrix (NPSM). Images 1, 2, and 3 represent the Au/NPSM composite structures after annealing at 50 °C, 200 °C, and 500 °C, respectively. Illustration from the article in MDPI
The peculiar state of the nanoparticles opens up not only an additional measurement for substance analysis but also novel properties. Thus, nanoparticles coated with chiral molecules are known to demonstrate specific mechanisms of cell penetration, depending on the type of enantiomer (L or D) situated on the surface. That, as well as the particles’ greater biocompatibility, might be of use for medical applications.
Apart from being easily implemented and affordable, the method proposed by the scientists makes it possible to create chiral ensembles with an area 1 million times the size of that achieved with electron-beam lithography or focused ion beam etching. The larger the area of the ensemble, the more analytes of a substance under study will interact with the surface. Such ensembles can be used, for example, to perform multiplex diagnostics involving microfluidic systems (the study of fluids in a continuous-flow mode) or produce labs-on-a-chip.
Additionally, the chiral ensemble can be studied using traditional circular dichroism spectrophotometers, whereas micronic structures obtained with lithographic methods require special-purpose optomechanical and optical equipment.
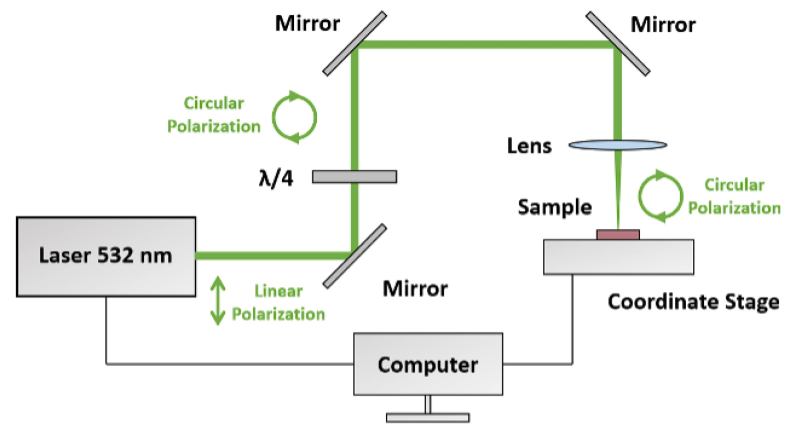
A schematic of irradiation of a racemic ensemble of gold nanoparticles in an NPSM. Illustration from the article in MDPI
Prospects
In the future, the researchers plan to switch their focus to more applied tasks. In partnership with their colleagues from the Skolkovo Institute of Science and Technology (Moscow), they intend to develop gas-sensitive devices for the detection various analytes (e.g., ammonia, ethanol, acetone, and methanol) for environmental uses, and together with experts in chiral plasmonics and photonics from Tel Aviv University (Israel), they are working on photochemical methods for the production of chiral nanoparticles.
This research received support from the Russian Science Foundation via grant No. 21-72-10098.
Reference: Anastasiia Sapunova, Yulia Yandybaeva, Roman Zakoldaev, Alexandra Afanasjeva, Olga Andreeva, Igor Gladskikh, Tigran Vartanyan, Daler Dadadzhanov, Laser-Induced Chirality of Plasmonic Nanoparticles Embedded in Porous Matrix. Nanomaterials, 2023.