Current methods for cancer diagnostics and treatment
There are two main methods used to treat cancer: radiotherapy and chemotherapy, each of which have their drawbacks. In radiotherapy, as tumors are exposed to radiation, so are the tissues surrounding them. During chemotherapy, patients receive intravenous injections of treatments that are toxic for actively dividing cells. However, such are not only cancer cells, but also the healthy cells and tissues of the body. That’s why the consequences of chemotherapy are most noticeable in those tissues where cells are renewed most often, such as hair and nails.
The commonly used cancer diagnostics methods also have their problems. For instance, sometimes MRI scanning can produce low-contrast images because metastases at the early stages can be very small, making them difficult to detect without special contrasting agents. The existing agents, at the same time, are not always efficient and have a toxic effect on the entire body.
Another approach to cancer treatment and diagnostics involves highly specialized nanoparticles with limited applications – they are developed specifically for each diagnostics or treatment method. Moreover, these particles cannot be combined in the body at the same time, as this will be even more damaging for the patient. This means that clinicians are forced to carefully choose one specific particle or agent, then introduce it into the treatment, and if it proves ineffective, switch to another one – but only after a specific amount of time. As a result, it extends the treatment, as well as lowers its overall efficiency.
Researchers at ITMO's Gene-therapeutic agents based on nucleic acids lab. Photo by Dmitry Grigoryev / ITMO.NEWS
Nanoparticles developed at ITMO
In order to address these problems, researchers from ITMO University aimed to combine several diagnostics and treatment methods: radiotherapy, magnetic hyperthermia, and MRI. As a result, they developed a multifunctional magnetic particle from zinc ferrite and manganese ferrite that can have several applications: serve in MRI diagnostics, as well as act as an agent in magnetic hyperthermia and a repeater in radiotherapy. Thanks to these properties, the new particle will provide a consistent, complex, and safe approach to cancer treatment and diagnostics.
In their article, the researchers demonstrate that the nanoparticles can serve as contrasting agents while working in two modes, T1 and T2. This bimodality yields more information during diagnostics. The nanoparticles’ contrasting properties were studied using a clinical MRI scanner.
The new nanoparticles can also find their applications in cancer treatment. In order to test their efficiency in radiotherapy, they were incubated with cancer cells from the large intestine for 24 hours in a Petri dish. After that, the cells were subjected to different doses of X-ray radiation. The researchers analyzed cell survival rates at different time intervals. It turned out that nanoparticles are most effective in destroying cancer cells at low doses of radiation: in this case, their efficiency increased by 40%.
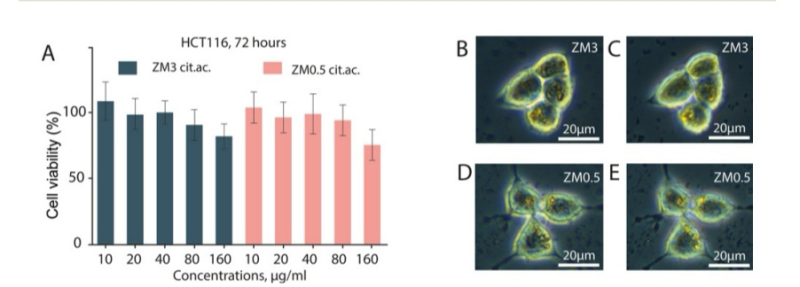
Left: cell survival rates depending on nanoparticle concentration. Right: analysis of nanoparticle absorption rates. Credit: pubs.rsc.org
Moreover, the nanoparticles heated up when placed into an external magnetic field, which means they can be utilized in magnetic hyperthermia. This type of treatment consists of raising the temperature of nanoparticles up to 42 degrees Celsius in just a few seconds via an oscillating magnetic field. In the process, cancer cells are killed, since their proteins break down at high temperatures, while healthy tissues remain unaffected. As a treatment, hyperthermia has the advantage of letting doctors target specific areas without the need to heat up their patient’s whole body.
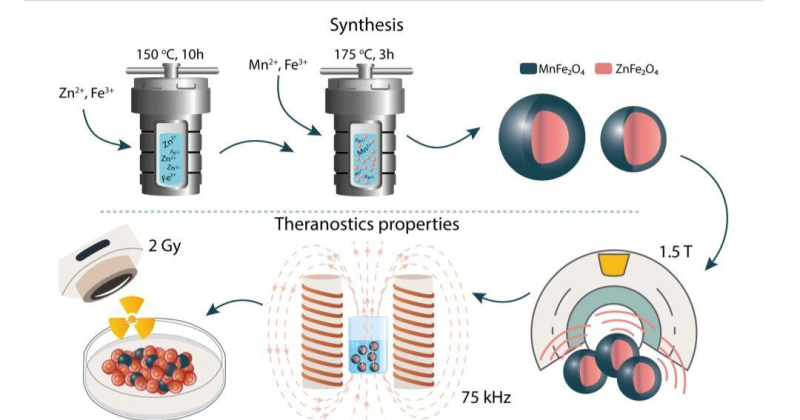
The image illustrates nanoparticle production stages. First, in the course of two-stage hydrothermal synthesis, the researchers acquired core-shell magnetic particles. Then, they analyzed the particles’ contrasting capacities, as well as their ability to heat up in the magnetic field and amplify X-ray radiation for diagnostics and treatment. Credit: pubs.rsc.org
How nanoparticles are made
The nanoparticles consist of two components: a zinc ferrite core and a manganese ferrite casing. These materials are easy to work with and synthesize, and their cytotoxicity is reasonably low. Furthermore, ferrites are superparamagnetic, which means they can be used in both MRI and hyperthermia.
In essence, the nanoparticles are made as a result of combining several molecules in a process called hydrothermal synthesis. It works a lot like pressure-cooking: the raw chemical ingredients are heated up inside an airtight vessel in order to achieve the extreme conditions required to synthesize the desired particles.
In the study, the composition, structure, size, and cytotoxicity levels (their compatibility with living cells) of these particles are described in detail.
What lies ahead
“Having already proven that our nanoparticles combine both diagnostic and therapeutic properties, we managed to increase the efficiency of magnetic hyperthermia and radiation therapy by 40%. They can also be used in T1 and T2 modes of MRI, which is simultaneously a blessing and a curse. On the one hand, our particles are very versatile. On the other hand, they do not produce as clear and precise images as mode-specific particles do. That is why we focused on understanding the inner workings of contrasting nanoparticles in just the T1 MRI mode. In the future, we plan to develop a new type of nanoparticles that will be tailored for T1,” shared Ilia Shabalkin, one of the study’s authors and a junior research associate at the Laboratory for Applied Materials for the Energy Sector.
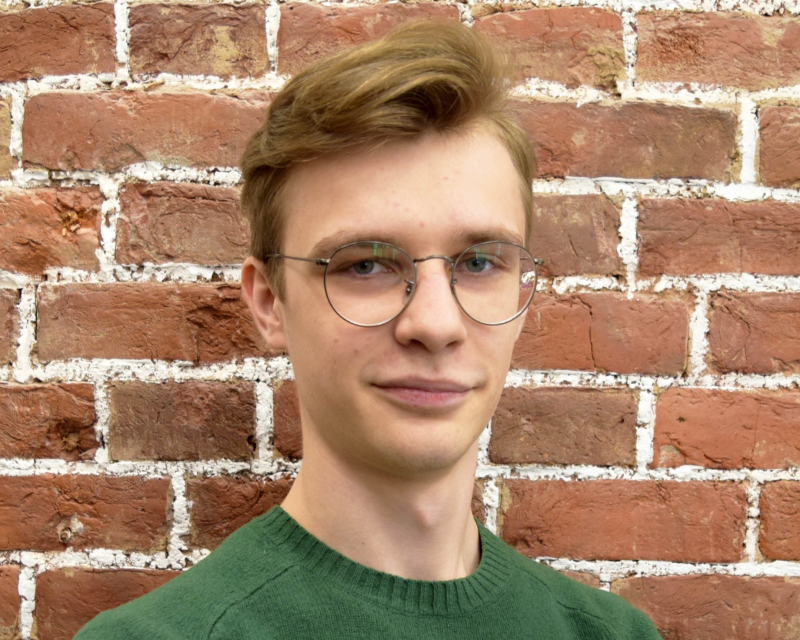
Ilia Shabalkin. Credit: scamt.ifmo.ru
Reference: Ilia Shabalkin, Alexey Komlev, Sergey Tsymbal, Oleg Burmistrov, Vladimir Zverevb, Pavel Krivoshapkin. Multifunctional tunable ZnFe2O4@MnFe2O4 nanoparticles for dual-mode MRI and combined magnetic hyperthermia with radiotherapy treatment (Journal of Materials Chemistry B, 2023).