Traditionally, the Nobel laureates are selected by the Nobel Committee for Physics, which consists of five members elected by the Royal Swedish Academy of Sciences. During the first stage, around 3,000 people nominate their candidates for the experts’ consideration. The Academy then chooses up to three candidates.
What is remarkable is that the Committee never announces the names of nominees, neither to the media nor to the candidates themselves. All nomination records are sealed for fifty years. However, sometimes the names of possible candidates appear in the media about a week prior to the announcement ceremony. For example, in the middle of September, Clarivate Analytics published its annual forecast of future Nobel Prize recipients. 17 world-class researchers made it to the Clarivate Analytics’s list. Among the possible Nobel Prize laureates in Physics were such researchers as:
David Awschalom from the University of Chicago (the USA) and Arthur Gossard from the University of California (the USA), for observation of the spin Hall effect in semiconductors;
Sandra Faber from the University of California (the USA), for pioneering methods to determine the age, size, and distance of galaxies;
Yury Gogotsi from Drexel University (the USA), Rodney Ruoff from Ulsan National Institute of Science and Technology (South Korea), and Patrice Simon from Université Paul Sabatier Toulouse III (France), for discoveries advancing the understanding and development of carbon-based materials.
The names of the Nobel laureates in Physics were announced in Stockholm on October 2, 2018. Among this year’s Nobel Prize winners are American scientist Arthur Ashkin, French researcher Gerard Mourou, and Canadian scientist Donna Strickland, who became the third woman to receive the Nobel Prize in Physics.
“The inventions being honored this year have revolutionized laser physics. Extremely small objects and incredibly rapid processes are now being seen in a new light. Advanced precision instruments are opening up unexplored areas of research and a multitude of industrial and medical applications,” says the official press release of the Royal Swedish Academy of Sciences.
Optical tweezers and their implications on medicine
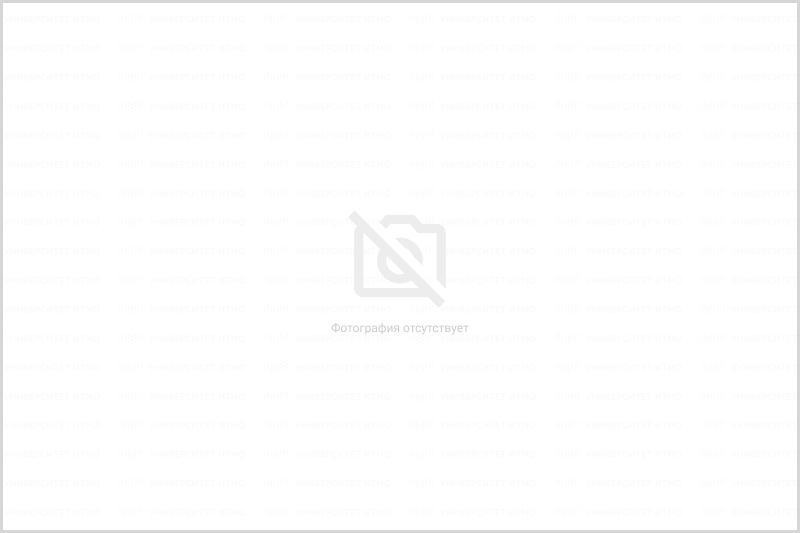
Arthur Ashkin was awarded the Prize “for the optical tweezers and their application to biological systems”.
Arthur Ashkin is an American scientist, a pioneer in the field of optical trapping, and a holder of 47 patents. Apart from that, he is also known for his extensive research in photorefraction, second harmonic generation, and non-linear optics in fibers.
The scientist invented optical tweezers that grab particles, atoms, viruses and other living cells with their laser beam fingers. This tool allowed Ashkin to make his old dream – using the radiation pressure of light to move physical objects, – come true. He succeeded in getting laser light to push small particles towards the center of the beam and hold them there. That’s how optical tweezers were created. In 1987, Ashkin used the tweezers to capture living bacteria without harming them. He immediately started studying biological systems. Optical tweezers are now widely used to investigate the machinery of life.
Alexander Shalin, head of ITMO’s International Laboratory of Nano-Opto-Mechanics, talks about the significance of Ashkin’s invention.
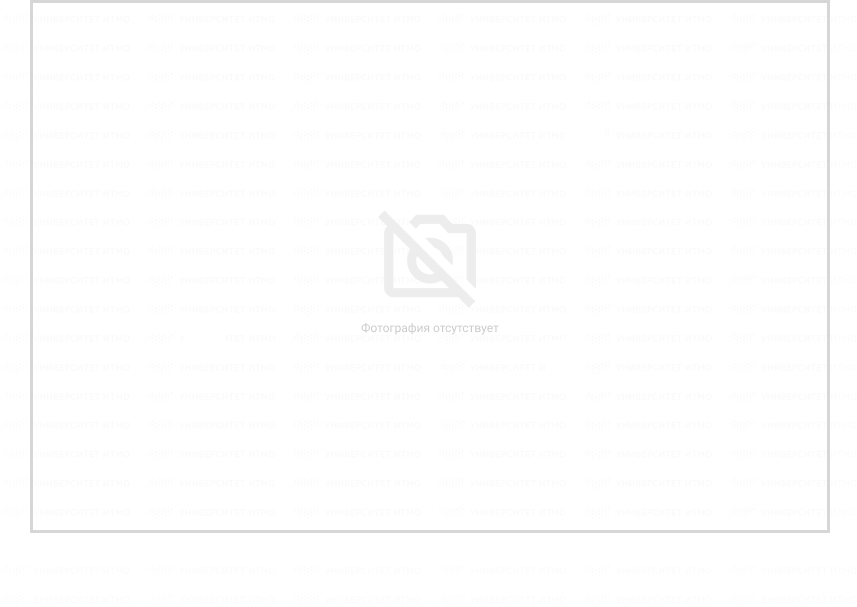
Was Arthur Ashkin the first to use laser radiation for manipulations with microparticles?
Broadly speaking, scientists attempted to do this before. For example, in as early as 1962 two Soviet scientists, Gurgen Askaryan and Vasily Moroz, published a Journal of Experimental and Theoretical Physics paper on displacing particles with laser beams. The crucial difference is that they proposed to do that by evaporating some of a particle’s fabric, which did cause it to accelerate, but at a cost of propelling its destruction. Ashkin’s optics-powered approach helped create a non-destructive particle manipulator.
How do the optical tweezers work?
A laser beam waist has the maximum radiation intensity, and that’s exactly how a gradient optical power acts: in the direction of the bigger intensity. So when a particle gets into a beam’s waist, it finds itself in a trap, which means that if the beam moves, the particle is bound to move with it. There are some exceptions to the rule, of course: in some cases, for example when a particle has a negative charge, it can, conversely, be pushed from the beam, but these conditions aren’t that feasible, so most practical applications of this technique draw on the first scenario.
Speaking of practical applications: how can we use this new discovery? And what does it mean for the future of science?
The invention of optical tweezers heralded a revolution in the field of microtechnologies: it gave us a non-pervasive way to displace extremely small objects, join them together, create different structures. Today, optical tweezers are widely applied, for example, in physics, to manipulate micro- and nanoparticles, atoms, and molecules and build structures on surface and in volume, which is made possible through the use of the so-called holographic tweezers able to grasp lots of particles simultaneously. In biology and medicine this type of particle manipulation is one of few of its kind that allow for a non-destructive capturing, displacement, and analysis of viruses and other cells
It can’t be overemphasized how promising a technology optical tweezers are, especially given the established trend for miniaturization of devices. I think that we have all reasons to believe that in the very near future, optical tweezers will be widely used for selective initiation of chemical reactions in micro-channels, with the aim of performing blood purifications or manufacturing artificial materials, for instance.
A novel technique for high-intensity, ultra-short optical pulses: from laser eye surgeries to a whole range of advanced physical systems
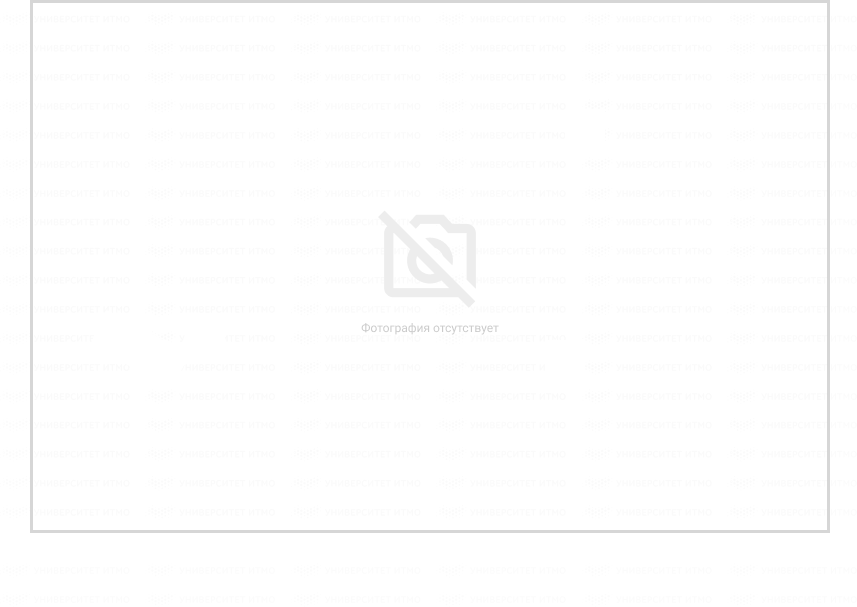
The other half of the 2018 Nobel Prize in Physics is to be awarded to professors Mourou and Strickland “for their method of generating high-intensity, ultra-short optical pulses”. Gerard Mourou and Donna Strickland are acclaimed French and Canadian physicists with a long research record. In 1985, they proposed an innovative solution for high-intensity, ultra-short optical pulses: chirped pulse amplification (CPA).
The scientists were first to succeed in creating a source of high-intensity, ultra-short optical pulses without destroying the amplifying material. Before this breakthrough, substantive pulse amplification had simply been unimaginable: even a single passing would have meant a total system breakdown due to the soaring intensity. Chirped pulse amplification method introduced a new generation of highly efficient femtosecond lasers with considerable power capacity that now serve as a basis for advanced systems included in both electronic devices and crucial laboratory units. But the technique’s versatility means that scientists often use it in novel, and often very surprising, practical ways.
Eduard Ageev, research associate at ITMO University’s Faculty of Physics and Engineering, discusses the new horizons the Mourou-Strickland discovery has opened in science.
How does the proposed method work?
Above all else, their technique is that of highly effective pulse amplification, in other words, of increasing pulses’ energy. Since we deal with ultra-short pulses that measure in femtoseconds, their direct amplification (for example, by topping up on the number of passes in a resonator) risks causing damage to optical elements and creating nonlinear effects such as self-focusing or breakdowns, which impedes the amplification process. That’s why the CPA method stipulates that before amplifying, the pulse stretches in time to reduce its peak power, amplifies, and then compresses, which is done through a system consisting of two diffraction grids.
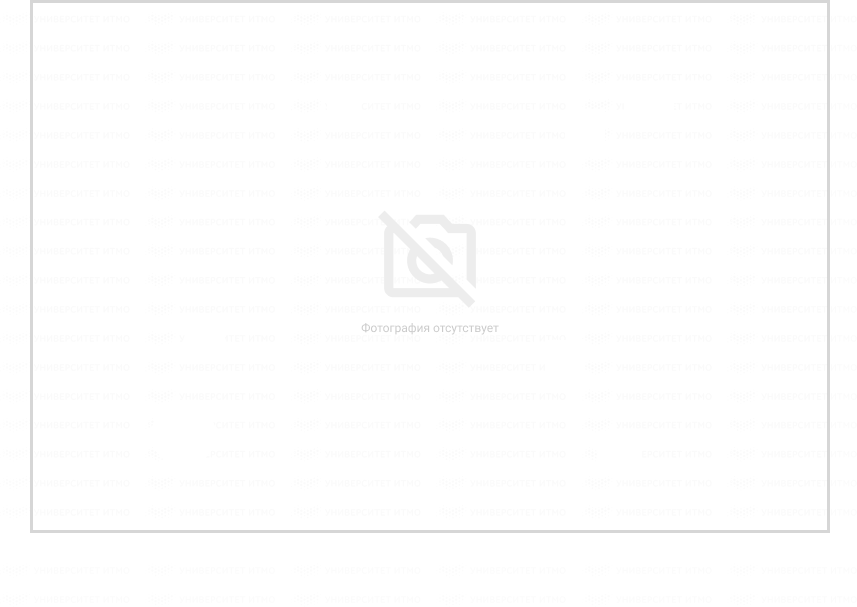
Which practical uses does the Mourou-Strickland technique offer?
There are a range of potential applications that include:
1) Production of ultraharmonics. The interaction between the high-intensity laser radiation and noble-gas atoms helps generate high-order harmonics of fundamental frequency all the way to vacuum-UV radiation and soft X-rays. Research on this spectral band is actively used in modern photolithography.
2) Eye surgeries (laser-based correction of vision, the so-called femto-LASIC).
3) Optical tomography and microscopy, studies in the field of non-linear quantum electrodynamics (including the relativistic interaction between radiation and matter).
4) Generation of supercontinuum (white light lasers).
5) Precision (micro- and nano-) processing of materials.
Today’s scientists continue their active exploration of these areas made possible by the Mourou-Strickland’s seminal discovery.
The Nobel Prize in Physics is one of the five Nobel Prizes established by the will of Swedish industrialist and inventor Alfred Nobel in 1895 and awarded since 1901. The first Nobel Prize in Physics was awarded to German physicist Wilhelm Röntgen in recognition of the extraordinary services he rendered by the discovery of the remarkable rays (or X-rays).
This award is administered by the Nobel Foundation and regarded as the most prestigious award a scientist can receive in physics. The rules require that the significance of achievements being recognized has been “tested by time”, which implies at least a 20 years lag between the discovery and the award.
The ceremony will take place in Stockholm on December 10, the anniversary of Nobel’s death. Four of the five Nobel Prizes are awarded in Stockholm, while the Nobel Peace Prize is awarded in Oslo. The Nobel Prize is worth 9 million Swedish crowns (more than $1.1 million).