Extracellular communication is largely responsible for the smooth functioning of the human body and its organs. Cells distribute nutrients and contribute to their synthesis, while also removing toxins and protecting the body from infections. When extracellular communication is disrupted, muscle and bone regeneration takes longer; at the same time, the body can develop various conditions such as Alzheimer’s disease. In order to develop treatments for these conditions, it is important to understand the mechanism of cell communication in detail.
One way in which cell communication occurs is via currents of calcium, sodium, and potassium ions that pass through the cell membrane into the extracellular space and back into the cell. Each type of ion is responsible for a specific function: sodium cations conduct nerve impulses, potassium cations regulate membrane potential, while calcium cations help contract muscles. When we feel pain or are under stress, for example, the body releases a substance to trigger cellular response. For instance, when norepinephrine (adrenaline) activates IP3 ion channels in a cell, this causes it to release calcium ions and contract, sending a signal to its neighbor cells. Next, in a domino-like effect, cells contract one after another, eventually contracting muscles.
There are several methods for studying ion currents. One of them involves inserting a microelectrode inside a cell; however, this can trigger the emission of specific proteins that will affect the measurement of ion currents. Other systems are based on growing artificial tissue and then creating breaks between cells – here, too, there is a possibility of damaging the cell. Researchers from ITMO’s Infochemistry Scientific Center have created a biocompatible system for detecting ion currents in the extracellular fluid that helps observe cells in their natural habitat without interfering with their activities.
“Our system is based on hydroxyapatite (a mineral that forms bone tissue). The platform itself consists of several Liesegang rings – concentric circles that form as a result of precipitation of a solution. We primarily grew cells on these rings. One way that cells communicate is through ion channels. We added norepinephrine, which activates calcium channels, in order to evaluate the pattern of signal distribution within rings and between them. The system’s second component, flexible ion-selective microelectrodes, were used to change the ion currents occurring in the cell. The registered wavelike signal redistribution after the activation of ion channels serves as proof of signal transmission between cells,” says Polina Zyrianova, the paper’s first author and an engineer at ITMO’s Infochemistry Scientific Center.
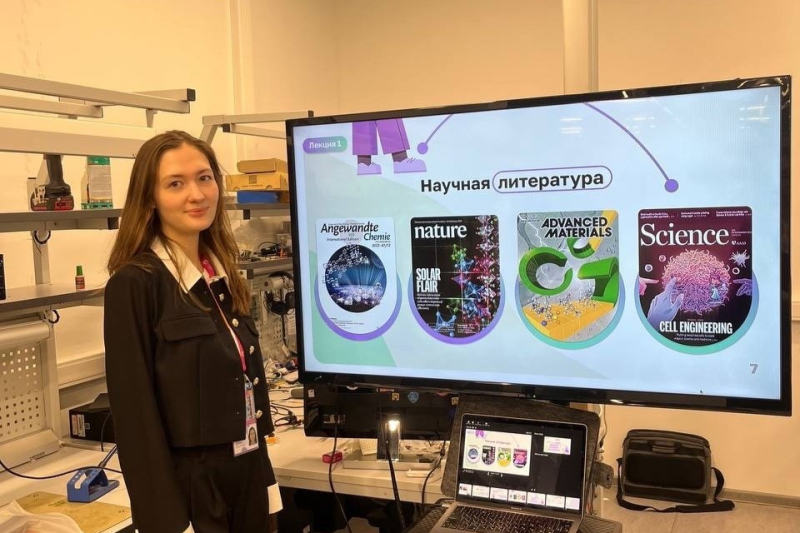
Polina Zyrianova. Photo courtesy of the subject
According to the project’s authors, the connection between rings resembles that between cities. Even though cells settle separately on the rings, they “sense” each other from afar and can transmit signals and even grow towards each other, even though they don’t have sense organs or a consciousness. During their work on the extracellular communication platform, the researchers also noticed the most active cell growth on the third hydroxyapatite ring; they discovered that this ring has the greatest piezoelectric response. The latter plays an important role in bone tissue regeneration – it transforms mechanical pressure into electric pulses that attract stem cells. Once on site, stem cells differentiate into osteoblasts and form new bone tissue or firm up the existing one.
For example, martial arts practitioners will have firmer bones than people who do not practice such sports. This would be the result of constant mechanical pressure and the related piezoelectric response. Studying the effect of piezoelectric response on extracellular communication can help the development of functional materials for targeted drug delivery systems.
“We have created a model biomimetic system that resembles a natural bone with a piezo-response. Additional substances can be introduced into the system to stimulate cell growth or release medicine. These studies can be beneficial for the development of implants that facilitate cell regeneration. We are planning to continue our research on re-establishing disrupted cell communication with the goal of designing a material that cells will recognize as ‘kin,’” adds Svetlana Ulasevich, one of the paper’s authors and an associate professor at ITMO’s Infochemistry Scientific Center.
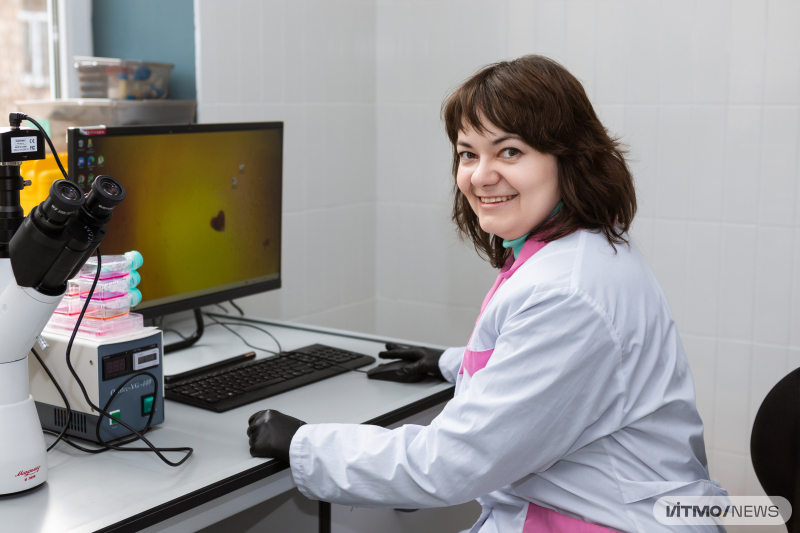
Svetlana Ulasevich. Photo by Dmitry Grigoryev / ITMO.NEWS
This research project was supported by the Russian Science Foundation (grants No. 21-13-00403 and No. 19-79-10244).