Soccer as a gateway to science
I first became interested in science when I was eight, and I began to explore nature more closely, identifying patterns, regular and recurring, in my surroundings. That was the beginning of my story with physics. I took joy in watching grass growing and looking for symmetry in flowers. I also learned about the concept of pi around that time.
It happened when I watched a soccer ball, which looked to be perfectly spherical, much like planets, as I subsequently discovered. Their likeness piqued my curiosity. I learned that planets have a diameter and radius from an astronomy dictionary that my parents gave me and sensed a link between the two – and that was pi. Later, I understood that a diameter-radius relation is not just a trait of planets but also soccer balls. That’s why I decided to do an experiment to determine the diameter and radius of a ball. The ratio was more or less equal to three, which was extremely close to pi. I suppose that was the first time that I was doing something resembling physics: I measured, observed, proposed a hypothesis, and then executed an experiment.
To get to know the world better, I opted to become a physicist and enrolled in the Faculty of Physics at the University of Seville in Spain.
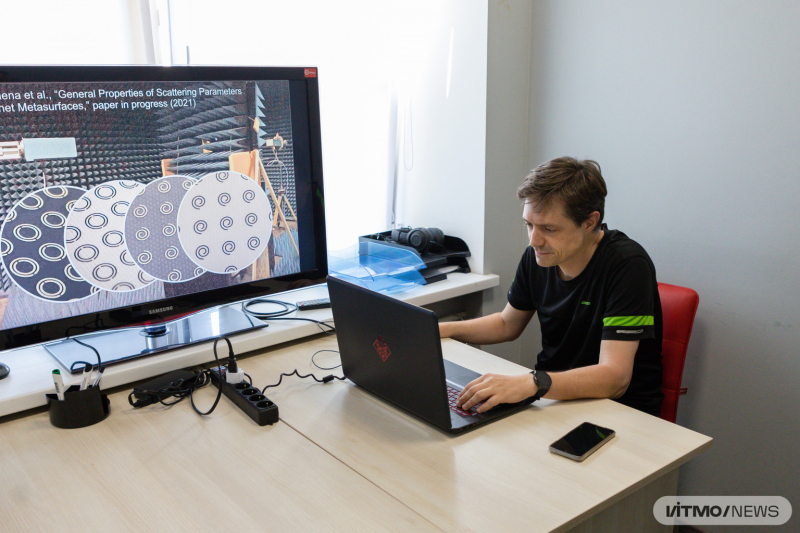
Juan Baena. Photo by Dmitry Grigoryev / ITMO.NEWS
Through conferences to collaborations
When I was a student, I was invited to participate in a grant project on metamaterials, which became relevant in science at that time. As the research community sought fresh ideas for the perfect focusing of electromagnetic waves, I did something similar as part of the microwave group at the University of Seville led by Prof. Ricardo Marques Sillero. Although I was excited about metamaterials, I chose to concentrate on electromagnetism, its spread and antennas – a field I’ve been in for years now.
Before getting my PhD in 2006, I was able to publish around 48 papers, both theoretical and experimental, primarily on the topic of periodic structures in electromagnetism.
I presented my findings at a number of international conferences. In 2008, for instance, Pavel Belov, the chief researcher at ITMO’s Faculty of Physics, invited me to the International Conference Days on Diffraction in St. Petersburg. Back then, we hadn’t worked with him yet, only met each other. That marked the beginning of my work with ITMO.
Four years later, I attended another conference on metamaterials in St. Petersburg. We teamed up with Pavel Belov’s group in 2014 and published a joint article a year later. Our investigation focused on self-complementary structures and metasurfaces. I was responsible for the theoretical aspect of the paper, and my colleagues from ITMO assisted with experiments. Working together boosted our relationship, which is still going strong today.
The International Conference Days on Diffraction. Credit: ITMO.NEWS
ICDs with wireless charging
I collaborated on several metasurface-related studies with ITMO researchers, some of which were centered around their refraction and focusing abilities, while others – on their capacity to absorb electromagnetic waves. At some point, my colleagues at ITMO’s Faculty of Physics, led by Polina Kapitanova, Stanislav Glybovski, and Mihail Petrov, received a megagrant for the project Wave Processes in Medical Systems and brought me on board. That was a significant step forward in our partnership.
One of the project’s goals is to design and develop a wireless charging system for medical implants, particularly implantable cardioverter-defibrillators (ICDs). An ICD is a device that is programmed to detect life-threatening heartbeats and prevent cardiac arrest. ICDs are normally powered by batteries, which, when running out, have to be replaced. But what if it was possible to charge ICD batteries without a surgical intervention?
However, numerous challenges must be addressed before such a system can be implemented, one of which is associated with electromagnetic waves inside the human body. The problem is that the human body consists of a great amount of water that absorbs electromagnetic frequencies. As a result, a wave can only penetrate a few centimeters, which is not enough to reach an implant with a sufficient level.
Say you want to carry out wireless energy transfer in a space where there are no obstacles such as biological tissue. It is quite simple to implement with two coils. If two charged coils are adjacent, they will interact. This phenomenon is called induction. In the presence of an impediment, however, the coupling between the coils will deteriorate.
The solution could be to convert an electromagnetic wave into an acoustic one. Unlike the former, acoustic waves may overcome biological obstacles and reach “the second coil,” namely an ICD. This kind of conversion requires a metalens, which is a thin lens made up of an array of vertical metamaterial nano-antennas. Metalenses capture an electromagnetic wave and transform it into energy that creates an acoustic wave. After that, the acoustic wave spreads in the human body and reaches the device. As acoustic waves cannot charge an ICD, the process has to be reversed within the body.
To that end, the system must consist of several components: a wearable device collecting the power of radiofrequency signals of the surrounding space and transforming it to ultrasonic waves; a wearable acoustic transmitter combined with a power accumulation system and a focusing metalens; and an implanted device incorporating an acoustic receiver.
Read also:
New Generation of Wireless Chargers Developed at ITMO: Here’s How It Can Change Your Life
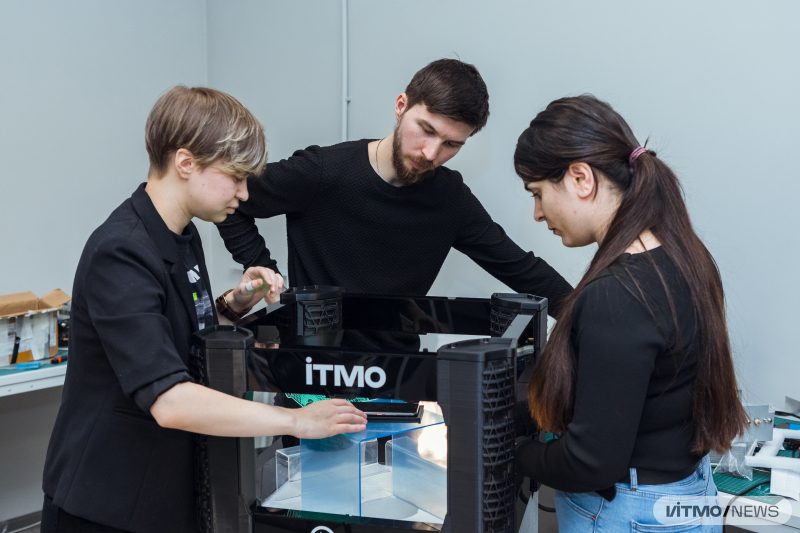
Members of the development team next to their wireless charger prototype. Photo by Dmitry Grigoryev / ITMO.NEWS
“Math is not easy”
The project includes four phases. The first step is to develop theoretical methods and tools that will help us describe how electromagnetic and acoustic fields interact with body tissues and what constraints may exist. The use of radio frequencies for MRI and wearable antennas and metalenses will be our next emphasis. Last but not least, we will study interactions between acoustic waves and biomedical equipment and put a system together.
I am engaged in several aspects of the project – theoretical ones for the most part. These are the development of numerical methods for focusing an electromagnetic field and impedance matching using metamaterials to reduce the reflection of an electromagnetic wave when entering the body.
My area of expertise is numerical simulation and formulas. After all, before conducting any experiment, we have to first validate our calculations and then create a model that is the closest to the original. As a result, the majority of the difficulties I encounter are mathematical in nature. Math is not easy, just like it was in school. However, talking to your colleagues who might provide you with advice even if they aren't experts in the field is a terrific method to conquer a problem.
Another option is to run a numerical simulation to determine what is not functioning. Once, it took me two weeks to find an error in my calculations. But had I not found it, I wouldn't have been able to proceed.
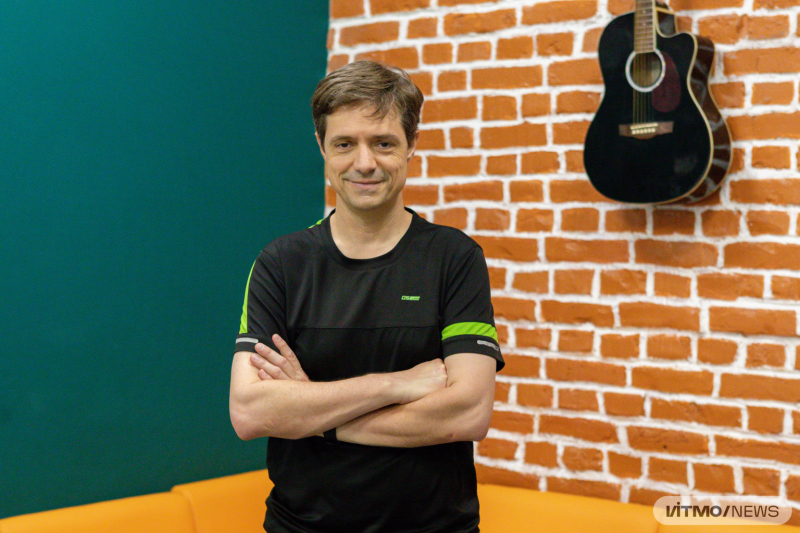
Juan Baena. Photo by Dmitry Grigoryev / ITMO.NEWS
Trending science
Wireless power transfer has been and still is a hot topic. Researchers are currently working to create miniature flat devices, like lenses, to control polarization, as well as focus, refract, and spread electromagnetic waves. As miniature lenses lose volume – vital for focusing and manipulating waves – they have to be made of metasurfaces. This way, the device will remain small and flat, and a metasurface will take on polarization control.
Metalenses can not only enable wireless charging for a range of implanted devices – they can also improve cell signal strength. To receive a signal, a smartphone must collect electromagnetic waves emitted by antennas located outside a building. But thick walls, which absorb and reflect a portion of the waves, may get in the way. But what if we developed a transparent metasurface that will receive a wave and refract it at a certain angle – all while remaining invisible to the user? A metasurface like that may be put on a window, allowing a wave to pass through and so improving reception.