Loud music at night or the repairs your neighbors have decided to do on the weekend: these are examples of acoustic noise and are of no interest to researchers – as opposed to random noise. To understand the difference between them, imagine a piano. Pressing a single key produces an acoustic wave of one tone, characterized by a set of frequencies. By adding a second key, we increase the number of tones and thus, frequencies. However, even if you press all the keys on a piano at once, it will still produce an acoustic wave with a finite number of frequencies. But if you imagine a musical instrument with an infinite number of keys that slightly differ in sound and frequency, and if you were to press them all simultaneously, you would produce not acoustic but random noise – this is what captivates physicists.
However, it can be challenging to create a random noise source that would be adjustable depending on the task. Researchers from ITMO’s International Research and Educational Center for Physics of Nanostructures and their collaborators from the Institute for Problems in Mechanical Engineering of the Russian Academy of Sciences have conducted a study of how non-stationary topological defects in a layer of liquid crystals can be used as a source of beneficial random noise.
Read also:
Good Chaos: How Chaos Theory Helps Scientists and Everyone Else
Liquid crystals find their applications in digital devices – for instance, in high-contrast TV displays. However, within these materials it’s also possible to incur electrodynamic instabilities – chaotic flows of liquid within the crystal when it’s subjected to electricity. This was the feature that captured the interest of researchers from ITMO.
“When voltage is applied to a liquid crystal, the liquid within it starts flowing chaotically. These flows form unpredictable non-stationary topological defects. From the outside, these flows look similar to moving fractal worms; but they are actually a great source of random noise with properties that can be adapted to various tasks. With the increase of voltage, the “worms” grow smaller and start moving faster. With machine vision and fractal analysis, we studied which values correspond to various topological effects, what happens to large- and small-scale fractal dimensions, and how one scale transforms into the other. Based on these dependencies, we can train a neural network to set the random noise generation parameters on request from an operator, and to be able to detect the result,” shares Dmitrii Shcherbinin, the paper’s first author, a senior researcher at a lab of the International Research and Educational Center for Physics of Nanostructures.
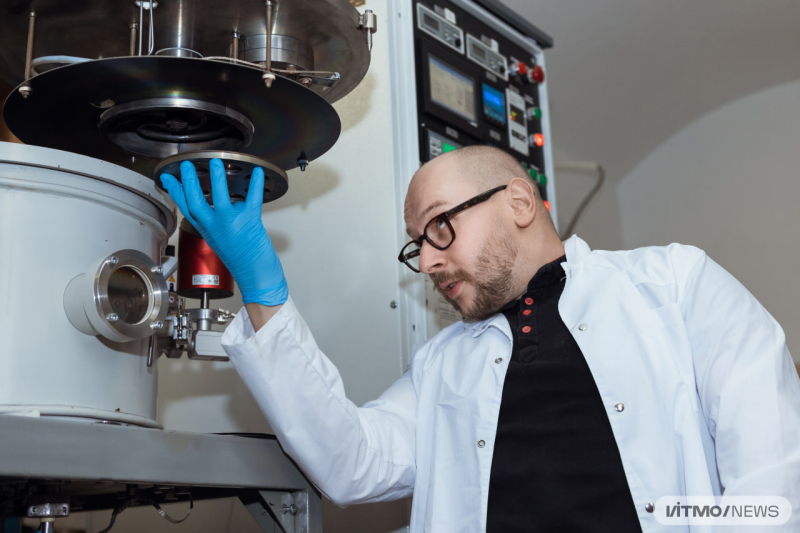
Dmitrii Shcherbinin installs a substrate inside a vacuum camera for the application of thin-film electrodes by sputter deposition. Photo by Dmitry Grigoryev / ITMO.NEWS
Regulated random noise generation can be useful in various fields. For example, it can serve as a generator of truly random, unpredictable numbers for more reliable data encryption and password generation in quantum cryptography and optimization. Additionally, random noise can be applied in quantum computing and phantom imaging. The latter allows for the acquisition of full-scale images using a single-pixel camera that is hundreds of times smaller than the smallest endoscopes currently in use for internal examinations of patients.
“A real-life case of a global optimization task may look like this: imagine that you are looking for a pair of boots at an online store, you’ve scrolled five pages down, and seem to have finally found it. However, that perfect pair will be on the tenth page, but you don’t know that yet. Random noise will cue you to keep scrolling till that perfect pair – and for a physical system, the cue will be to take on the extreme value, a global minimum or maximum. In phantom visualization, random noise helps turn a single-pixel camera image into a full-scale one. The process is similar to the game Battleship: random noise, like a player, picks a random square in the opponent’s field. The opponent says if their ship was hit or not – or, in the case of the camera, if the pixel is colored. At the end of the game, you have a map with sunken ships – or a sum of all pixels that forms the silhouette of an image in phantom visualization,” explains Georgii Demianchuk, one of the paper’s authors, a fourth-year student at the International Research and Educational Center for Physics of Nanostructures.
In the future, the researchers are planning to study the nature of observed topological defects in further detail.
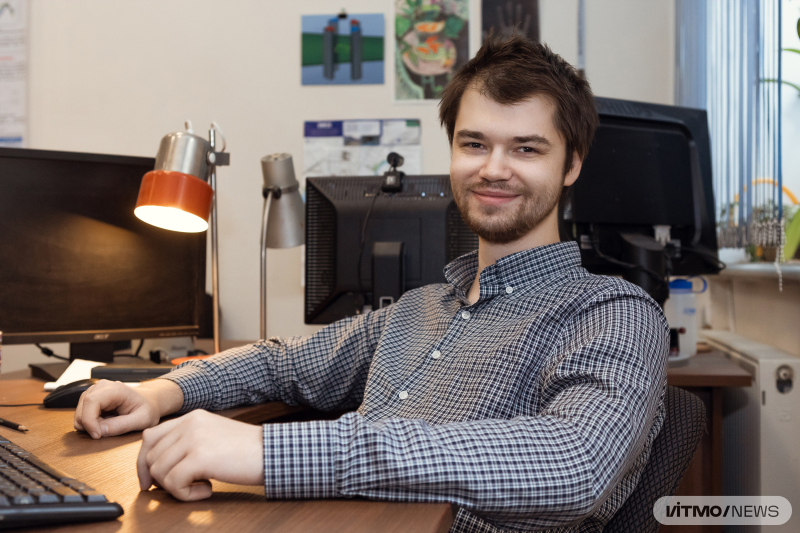
Semyon Rudyi. Photo by Dmitry Grigoryev / ITMO.NEWS
“We want to run a series of experiments to understand how some defects affect others; in other words, to statistically test which “worms” produce the best completely random number sequences, and how they can be adapted for various tasks,” adds Semyon Rudyi, one of the paper’s authors and the head of a lab at ITMO’s International Research and Educational Center for Physics of Nanostructures.
This research project is supported by the Russian Science Foundation (grant No. 24-79-00225).