Quantitative vs qualitative
ITMO’s Faculty of Physics has long been developing new devices for MRI, such as amplified antennas and receiver coils that help produce more homogeneous and detailed MR images by amplifying the signal. Within this Megagrant project, the researchers are focusing instead on developing a brand new approach to producing and analyzing MRI data.
According to David Bendahan, visual data currently is the primary source of information in diagnostics. Clinicians can identify the size of an inflammation or tumor and its location, but this is not enough to assess the course of a disease or the efficiency of treatment. However, if instead of relying on visual data alone they would be able to work with exact numbers, this will qualitatively change the approach to diagnostics and treatment.
“These days, medical specialists rely on strictly quantitative methods in MRI analysis – they look at black-and-white images and have only the coloring of different areas to guide them. For example, inflammations appear as patches of white that can be very bright on one scanner and dimmer on another. If these scans are done on different days, an incorrect conclusion may be made that the inflammation was stronger and has now decreased – all because of the different contrast in these images. Numbers are a whole other matter – they don’t change and are always precise. If therapists had access to quantitative indicators and data on the inflammation, then they would be able to say for sure if it grew stronger or weaker,” says David Bendahan.
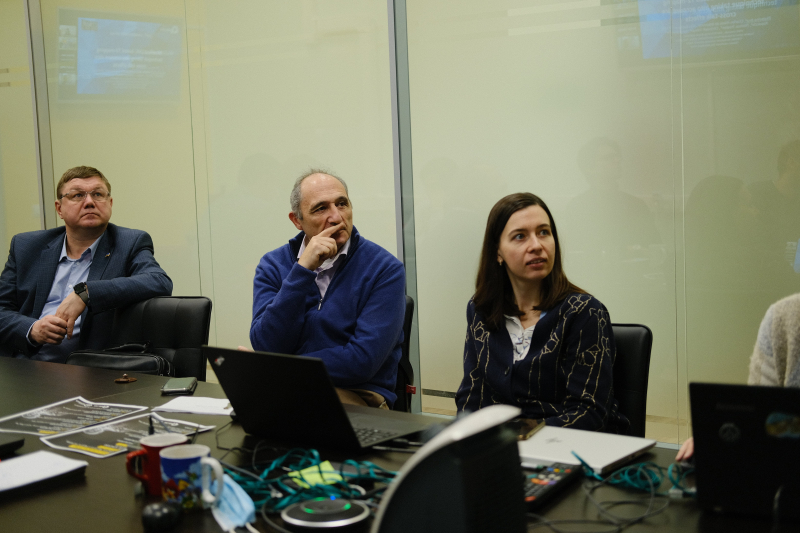
David Bendahan's workshop at ITMO. Photo by Liliya Kichigina, ITMO's Faculty of Physics
At the core of such quantitative MRI approaches are machine learning and artificial intelligence. And even though these methods aren’t new, their applications in MRI are still far from being introduced into practice. It is quite a non-trivial task to extract precise numerical parameters from visual data and it requires not only new tools and analysis algorithms, but also new imaging approaches that would result in more homogeneous and detailed scans.
“In order to perform a quantitative analysis, we need high-quality raw data with a low level of noise and without any additional distortions. But in practice these two requirements contradict each other – we have to increase the magnetic field to improve the signal-to-noise ratio and a stronger field leads to more distortions in the form of areas with none or weaker signal. In this project, we will be looking for a compromise for the classic scanner configuration, as well as applying our metamaterials- and ceramics-based devices to eliminate this contradiction between too much noise and the homogeneity of resulting images. And if not eliminate, then at least move it into stronger fields, which aren’t yet used in clinical practice,” adds Mikhail Zubkov, a researcher at the International Laboratory of Applied Radioengineering.
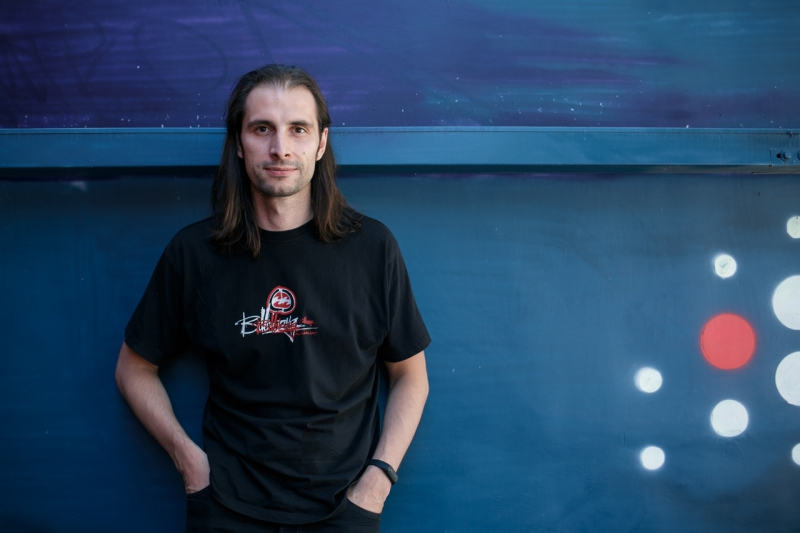
Mikhail Zubkov. Photo by ITMO.NEWS
MRI for challenging cases
Another area of interest for the Faculty of Physics are scans of hard-to-access organs, such as the liver or the heart, or body parts with a complex structure, such as wrists. Over the first year of working on the project, the researchers focused on producing high-quality images of hands and wrists. Dr. Bendahan explains that wrists have a complex anatomy and very delicate and small joints, which makes it challenging to acquire their images and even more so to analyze them. However, for certain conditions, such as arthritis and rheumatism, it is crucial to monitor what happens to joints and if their cartilage has thickened.
“We aimed to create a method for identifying the thickness of joints and cartilage, as this kind of information could really come in handy when treating hand diseases. We’ve developed an algorithm based on AI that can precisely identify the thickness of various segments of wrists and their joints based on a simple 2D MR image,” explains David Bendahan.
Such indicators are referred to as biomarkers and they are specific for every illness. In case of rheumatism, it’s cartilage thickening in wrist joints. The researchers are planning to learn to use similar biomarker-based quantitative methods for liver and heart diseases.
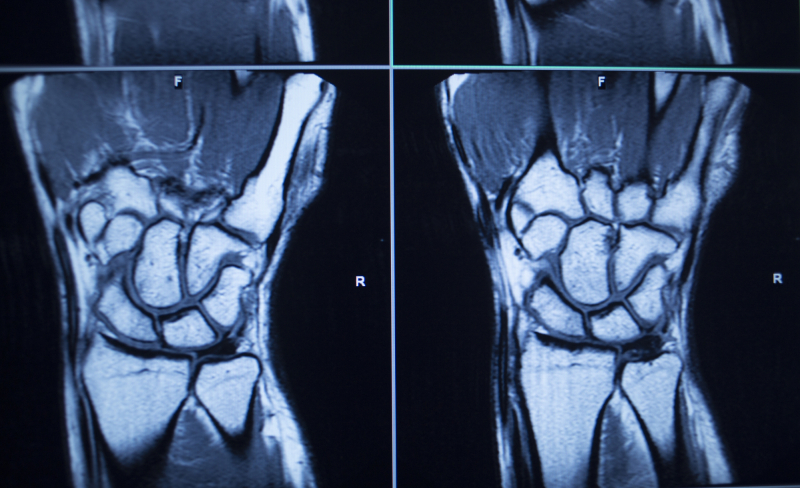
An MRI scan of the wrist. Credit: depositphotos.com
“Specialists have long known about the quantitative biomarkers for many diseases of the liver and the heart, such as, for instance, the percent of collagen-containing tissue in a liver sample. However, the very need to obtain a sample makes this biomarker hard to acquire, as well as potentially dangerous for the patient. That’s why now researchers are looking for non-invasive biomarkers that are mostly acquired using various diagnostic procedures (MRI, CT, ultrasound imaging) or laboratory tests (such as that of the blood serum). For instance, the controlled attenuation parameter (CAP) for the ultrasound, which is completely non-invasive, is used to diagnose fatty liver disease,” explains Mikhail Zubkov.
Networking is key
One of the goals of this megagrant project is to improve the communication between scientists and clinicians. Dr. Bendahan admits that it’s not a simple task because it can be hard for experts in completely different fields to see eye to eye with each other. However, such collaborations are necessary in order to achieve new scientific solutions to be implemented into the daily healthcare practice.
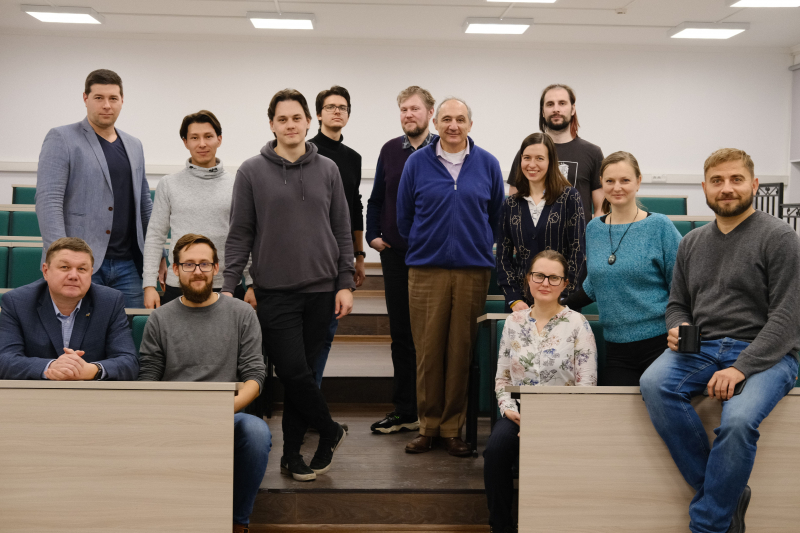
David Bendahan's workshop at ITMO. Photo by Liliya Kichigina, ITMO's Faculty of Physics
“Even before securing the Megagrant, the team of the Faculty of Physics had experience in developing MRI devices, but they had only a few connections with clinical specialists. This new project is a unique opportunity to have clinicians on board and to strengthen the links between physicists and medical staff. We have already welcomed several specialists from the Almazov National Medical Research Centre on the project. In the future, we are planning to grow this into a nation-wide collaboration,” concludes David Bendahan.