Every year, contest organizers honor the authors of the best graduation papers in Russia. In 2022, the jury awarded the first prize to Anastasia Olkhova and Artem Orlov for their projects in photonics and optoinformatics. This isn’t the first time ITMO students demonstrated great results at the contest. Last year, the university's young scientists from the Faculty of Photonics were awarded the first prize for their achievements in the field, as well.
“Our programs are developed in line with the scientific activities of ITMO’s International Research and Educational Center for Physics of Nanostructures. Last year, the Physics and Technology of Nanostructures Master’s program celebrated its tenth anniversary. Since its launch, we have achieved many heights. Many of our students decided to pursue their PhDs at ITMO, and some even got postdoc positions at the world’s leading universities. For instance, our student Maria Mukhina, who earned her Master’s and PhD at ITMO, is now working at Harvard University. What’s more, our students often receive presidential scholarships from the Russian government,” says Anna Orlova, the head of the aforementioned educational programs, a leading professor at the Faculty of Photonics, and a chief researcher at the International Research and Educational Center for Physics of Nanostructures.
Even though both of the winning graduation papers are devoted to quantum dots, they study the phenomenon from different angles. ITMO.NEWS talked to this year’s contest winners to gain more insight into their projects.
Anastasia Olkhova
Master’s graduate 2021, now a first-year PhD student at the Faculty of Nanoelectronics
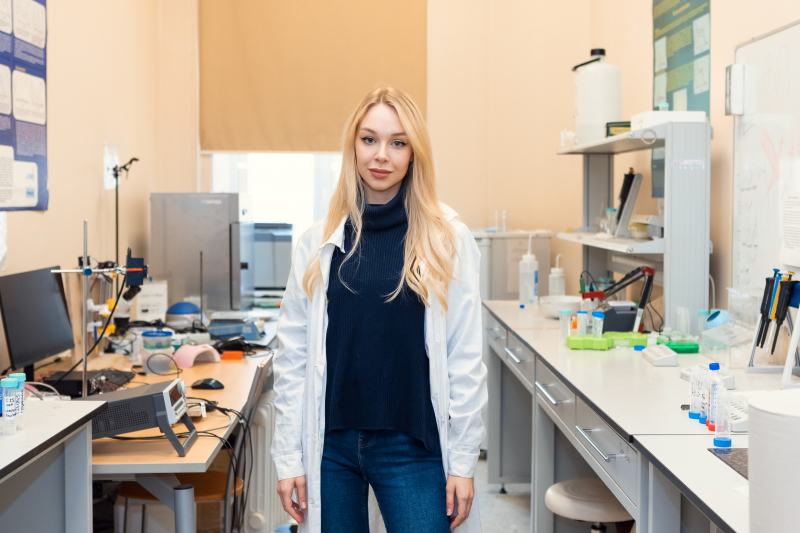
Anastasia Olkhova. Photo by Dmitry Grigoryev / ITMO.NEWS
On graduation thesis
I’ve explored the photoluminescence kinetics of CuInS2/ZnS quantum dots – ternary quantum dots used in various fields of photonics. The CuInS2 dots are primarily seen as a potential alternative to toxic QDs, which contain cadmium or lead. CuInS2/ZnS dots possess a broad photoluminescence spectrum that can be adjusted during the synthesis process or by changing the size, shape, and composition of the particles. They also have a high optical absorption coefficient and a high probability of radiative recombination, which makes them scientifically appealing for researchers engaged in biology, photovoltaics, and sensorics. We decided to study the ternary dots due to the scientific uncertainty about the processes underlying the radiation of these types of nanocrystals. In particular, we wanted to find a physical explanation for the broadening of their photoluminescence band.
On choice of topic
Before joining ITMO, I studied diamond-like nanofilms at St. Petersburg State University. Looking for laboratories working with nanomaterials, I came across ITMO's International Laboratory “Hybrid Nanostructures for Biomedicine.” What I loved most about the lab is that it deals with physics, chemistry, and biology and that its researchers conduct cutting-edge research at the intersection of different fields. Since Dr. Orlova is the head of my laboratory, I chose her as my supervisor and she recommended this topic to me.
During my first year, I was engaged in a different field. I was studying reactive oxygen species generated using hybrid nanostructures of thermally annealed titanium butoxide and cadmium selenide quantum dots. After some time, we decided to switch to ternary quantum dots and make our research more fundamental and extensive. All in all, we indeed produced a voluminous work and that’s probably what helped me win the contest.
Results and prospects
We strived to figure out what caused a noticeable increase in the width of the luminescence band of the chalcogenide-based quantum dots when compared to traditional A2B6 quantum dots, which include well-studied CdSe/ZnS quantum dots. To do so, we examined the kinetics of photoluminescence depending on the wavelength of the detected luminescence, the average distance between the quantum dots, and ambient temperature changes. As a result, we came to the conclusion that the broadening may be caused by the presence of both excitonic and defect-induced luminescence in chalcogenide-based quantum dots.
Our findings revealed that as the average distance between QDs decreases, the effects of resonance energy transfer begin to appear, thus affecting short-wavelength photoluminescence bands. It was also found that a temperature rise results in a rapid decrease in photoluminescence intensity, which goes hand in hand with the shift of spectral maximum and the broadening of the band. We found that the cause of the occurring physical processes lies in the thermal expansion of the quantum dots’ crystal lattice. That was an important insight that we also managed to demonstrate experimentally. The results obtained allow us to continue our research and eventually improve our knowledge of nanoscale semiconductors. Potentially, our discovery can pave the way for next-gen solar cells, LEDs, and medications.
Artem Orlov
Bachelor’s graduate 2021, now a first-year Master’s student at the Faculty of Photonics
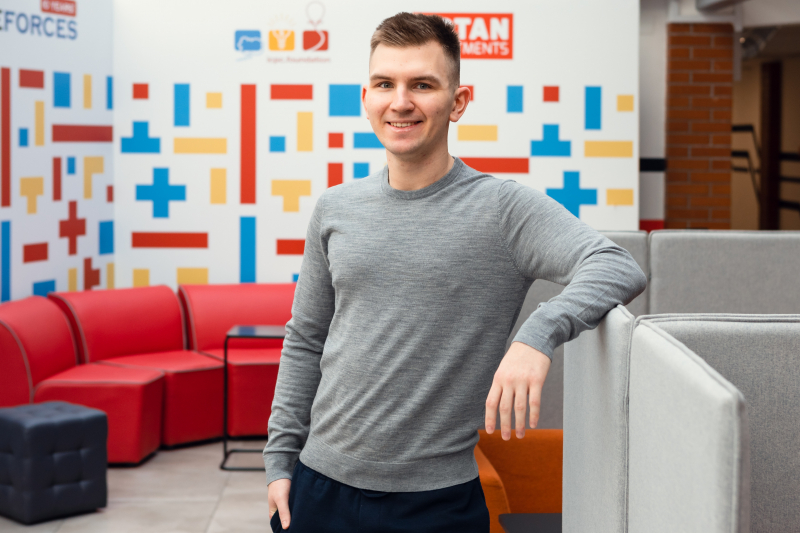
Artem Orlov. Photo by Dmitry Grigoryev / ITMO.NEWS
On graduation thesis
My graduation thesis was about twisted bilayer graphene quantum dots. Graphene is a two-dimensional material that is made of a single layer of carbon atoms tightly bound in a hexagonal honeycomb lattice. Although the material has been studied since the mid-20th century, there were no experiments until fairly recently when scientists Konstantin Novoselov and Andre Geim successfully produced the material and were awarded the Nobel Prize in 2010 for their achievements. Their discovery set a trend for studying graphene and structures based on the material both theoretically and experimentally.
One of such structures is twisted bilayer graphene, the two layers of which are rotated with respect to one another. Unlike traditional graphene, this graphene-based structure exhibits chirality or, in other words, optical activity, meaning that it absorbs left- and right-circularly polarized light differently. A unique feature of such material is that its optical activity is remarkably strong for its atomic thickness. Our scientific interest was in the optical properties of graphene-based quantum dots of various shapes.
On choice of topic
Having graduated from the Presidential Physics and Mathematics Lyceum No. 239, I was determined to study physics because I was extremely passionate about the field. As a result, I got into ITMO. I knew for sure that I’d be doing theoretical physics, so I asked Ivan Rukhlenko to be my supervisor. Halfway through my second year, I began to do term papers jointly with ITMO graduate Nikita Teplyakov, a PhD student at Imperial College London and a junior researcher at ITMO’s International Research and Educational Center for Physics of Nanostructures. Since both my colleagues were engaged in the study of graphene, I acquired an interest in the topic, too. I wanted to do what I enjoyed most – that is, dealing with theory and solving complex tasks – no matter the topic. By the end of the second course, I had already begun studying the material and when I was in my third year, I moved to bilayer graphene. In December, we went full force on our research. My graduation thesis combined both the results of our joint research plus further studies.
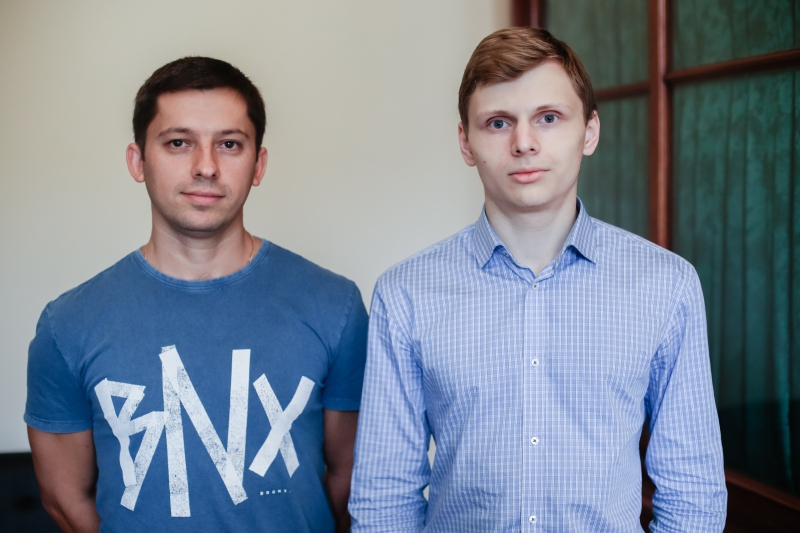
Ivan Rukhlenko and Nikita Teplyakov. Photo by ITMO.NEWS
Results and prospects
There is such a parameter called the dissymmetry factor, which measures the degree of chirality of various structures, meaning how differently they absorb light. During our research, we learned that for quantum dots made of twisted bilayer graphene, this parameter reaches one hundredth and exceeds the dissymmetry factor of experimentally obtained quantum dots by about two or three orders of magnitude. Once we manage to obtain such quantum dots experimentally, they will outdo the existing ones, including semiconductor and twisted quantum dots.
“These nanostructures hold great promise for next-gen functional devices and materials due to their unique optical, electronic, and photovoltaic properties associated with the quantum confinement effect. In his paper, Artem demonstrated that the electronic and optical properties of twisted bilayer graphene quantum dots can be controlled by changing not only the size and shape of the dots but also the rotation angle. These unique features make such quantum dots highly attractive for application in chiral nanophotonics. What’s more, graphene-based quantum dots can be used for bioimaging, biosensing, cancer therapy, targeted drug delivery, as well as in photodetectors and solar cells. Their high dispersibility in organic and aqueous solvents, excellent thermal- and photostability, and cytocompatibility make them great probes for identifying cancer cells. In turn, their photothermal and photodynamic properties (heat release and generation of reactive oxygen species upon irradiation) provide for a high therapeutic effect on cancer,” concludes Ivan Rukhlenko.
Right now, we’re still working on chirality, however, we also study the phenomenon using other structures. Our top priority is to find an environment that would make the efficient transmission of information between optical signals possible. This is our way to facilitate the transition to all-optical computing. Electronic processing devices are slower than optical ones; however, the latter require a special medium since photons can’t interact with one another in a vacuum. After six months, our calculations helped us realize that one medium won’t be enough to produce such an effect, so now we’re working on a different system.